LC8. Highs and Lows Over the Past 750 Million Years
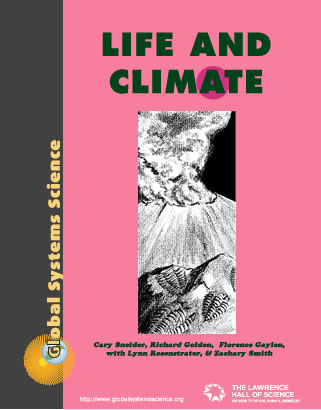
Chapter 8
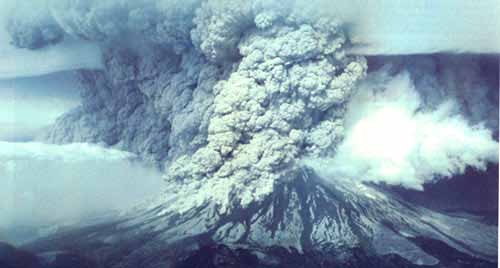
Volcanoes that occur near the edges of continents are called strato or ash volcanoes.
Volcanoes like Mount St. Helens were responsible for major changes in past climates because of the important role they played in the long-term carbon cycle.
While they sometimes produce lava, they are more likely to produce huge quantities of hot ash and gases, especially carbon dioxide gas. Where does the carbon dioxide come from? Where does it go? and Why is that important? The answers to these questions are related to the long-term carbon cycle, which provides a foundation for understanding why Earth’s climate has varied, and how those highs and lows in temperature have affected the evolution of life.
I. Earth’s Thermostat: the Long-term Carbon Cycle
Venus has a thick atmosphere that is almost all carbon dioxide. With the Sun’s heat trapped by the dense atmosphere, the surface of Venus is hot enough to melt lead. In contrast, Mars has a very thin atmosphere, and it is a cold planet. Neither Venus nor Mars has an ocean. Life as we know it doesn’t exist on either planet.
Earth’s atmosphere is thinner than the atmosphere of Venus but thicker than the atmosphere of Mars. Our air has a small amount of carbon dioxide (about three hundredths of one percent). Earth also has an ocean. Our atmosphere and ocean work together, acting as a sort of thermostat that keeps the temperature of our planet from getting too hot or too cold for life.
Global systems scientists generally agree on a theory about how the ocean interacts with the atmosphere and the solid crust to keep Earth’s temperature within a narrow range. Over the years, details of the theory will surely change as new information and ideas come to light. But here is a summary of the theory—called the long-term carbon cycle.
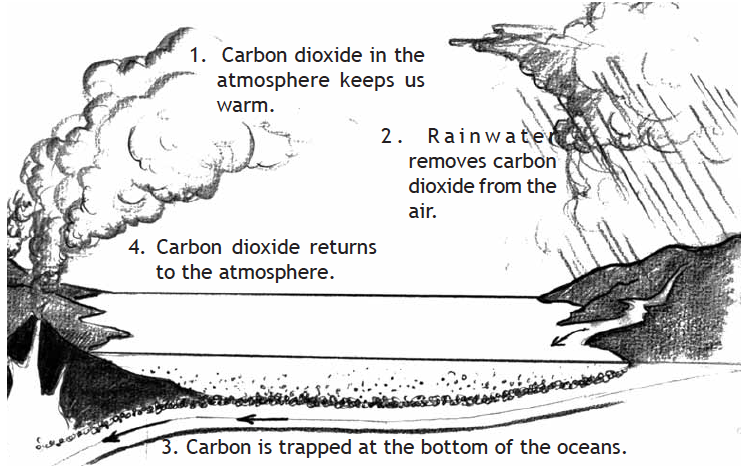
1. Carbon dioxide in the atmosphere keeps us warm. The concentration of carbon dioxide in the atmosphere today is about 350 parts per million. This level makes the average global temperature some 33°C warmer than it would be without carbon dioxide. This is because carbon dioxide and certain other gases in the atmosphere absorb infrared (heat) energy that would otherwise escape Earth’s atmosphere into space. This trapping of heat is called the greenhouse effect. Carbon dioxide is the major greenhouse gas.
2. Rainwater removes carbon dioxide from the air. As droplets of rainwater fall through the air, carbon dioxide is dissolved in the rain, forming dilute carbonic acid. When the rain reaches the ground, the weak acid dissolves and combines with minerals in rocks.
3. Carbon is trapped at the bottom of the oceans. Dissolved minerals flow down rivers to the ocean where they combine with other chemicals and form solid particles of carbon-containing rocks. Some of the minerals are incorporated into the shells and skeletons of living organisms. These carbon-containing solids eventually settle to the seafloor. These processes remove carbon dioxide from the atmosphere, reducing the greenhouse effect and cooling the climate.
4. Carbon dioxide returns to the atmosphere. Remember the theory of seafloor spreading? New volcanic rocks push upward at mid-ocean ridges where they solidify and form a wedge of new material. The new material pushes crustal plates away from the ridge center. These huge plates, along with the limestone on the ocean floor, move toward the continents. Reaching the continents, the crustal plates are pushed down, or subducted, into the mantle. When the subducted rocks reach a depth of 100 km or so, they melt, and the trapped carbon is released in huge bubbles of hot carbon dioxide and other gases. The gas bubbles and particles of pulverized rock rise to the surface and explode. The picture of Mount Saint Helens at the beginning of this chapter shows a huge cloud of ash—pulverized rock from the explosion—blasted into the air. Although carbon dioxide gas is invisible, it is an important part of the blasted material.
Each individual atom of carbon goes through steps 1, 2, 3, and 4 again and again, continuing the long-term carbon cycle. Happily for us, Earth will never get as cold as Mars. Mars has no drifting continents, so most of its carbon dioxide has either been ejected into space or is trapped in rocks. With no oceans, Mars has no long-term carbon cycle to return the trapped carbon to its atmosphere.
On Venus we find the opposite situation. Huge amounts of carbon dioxide exist in Venus’s atmosphere. Since Venus has no ocean, it never rains. With no rain, carbon dioxide remains in the atmosphere and keeps the planet at a constant high temperature.
II. Highlights of Earth’s Climate History
If the long-term carbon cycle were to operate smoothly, cycling exactly the same amount of carbon dioxide between the atmosphere and ocean every year, the climate would stay the same all the time. There would be no highs and lows. However, that is not the case. From time to time events occur that tip the balance so that carbon dioxide levels in the atmosphere are reduced or elevated. If an unusually large amount of carbon dioxide is locked on the ocean bottom, the planet will cool. Eventually, the rocks on the ocean bottom are forced down into the mantle, where they melt and release carbon dioxide back into the atmosphere through volcanic action. The result of these changes has been swings in the average global temperature, sometimes lasting millions of years. A history of Earth’s climate over the past half billion years is shown in the illustration.
The difference in average global surface temperature has ranged from 10°C warmer than it is now, to 6°C cooler than now. Although this doesn’t seem like much, the difference in temperature corresponds to a huge difference in regional climates. During the warmest periods, the climate on nearly all the continents was warm and humid. During the coolest periods, huge ice sheets covered much of the habitable land areas. When did the warmest and coolest periods occur? What was life on Earth like during those periods? Why were some periods warmer than others? These questions will be explored in the remainder of this chapter.
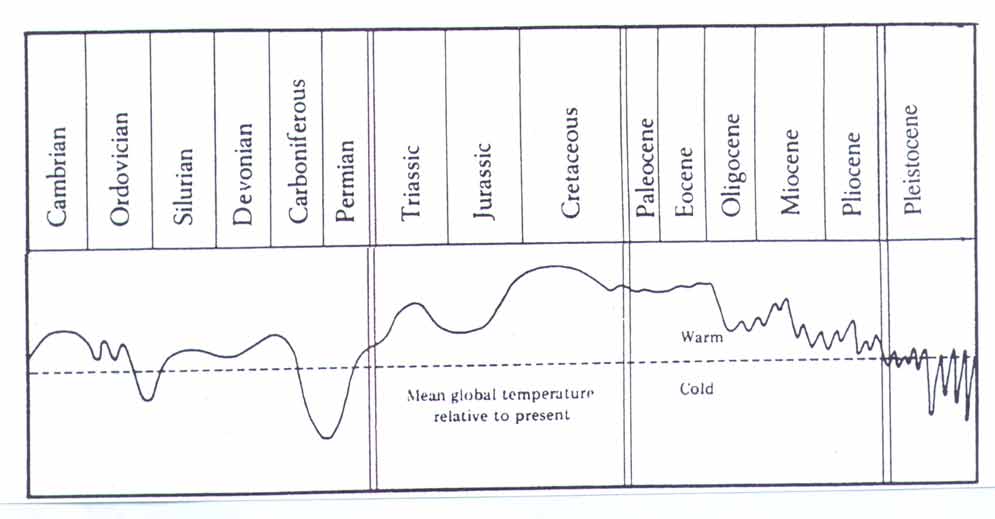
III. Climate Near the End of the Proterozoic Eon
(750 million to 580 million years ago)
Glaciers are slow-moving “rivers” of ice that grind away bedrock. As glaciers move and melt, they leave behind clear signs of where they’ve been, since they deposit clay and rocks of all sizes in heaps in their path. One of the most remarkable findings of recent years is evidence of glaciers in layers of rock from the end of the Proterozoic eon. In fact, it appears that as many as four extremely cold periods, or ice ages, occurred between about 750 million and 580 million years ago—just before complex life evolved.
In order to understand what caused those ice ages, it is important to know how Earth’s continents change over hundreds of millions of years. You may recall that about 200 million years ago all of Earth’s continents were joined together in one huge land mass called Pangaea. Pangaea was just the most recent supercontinent. Earlier, the continents were separated, and before that they had been joined together into another supercontinent called Rodinia.
According to a theory described by Paul F. Hoffman and Daniel P. Schrag in Scientific American (January 2000), the ice ages of the Proterozoic eon started about 770 million years ago, when Rodinia broke into smaller continents, which were grouped near the equator. Each land mass was surrounded by ocean. Since more ocean surfaces were next to land surfaces in warm tropical waters, there was an increase in rainfall. This meant that more carbon dioxide in the atmosphere was dissolved by the rain, more continental rocks eroded, and more and more carbon was stored in rocks on the ocean floor. With less carbon dioxide in the atmosphere, cooling began, and ice began to build up at the poles. The additional ice reflected sunlight, making the planet even colder. Within 1,000 years, the temperature was down to -50°C. Earth was in a deep freeze, with its oceans iced-over to the depth of a kilometer. The authors of the theory have called this condition the “snowball Earth.”
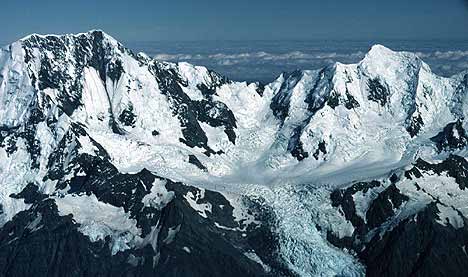
Photo courtesy of Thomas Lowell, University of Cincinnatti
How did our planet emerge from its deep freeze? According to Hoffman and Schrag, when the planet was completely frozen, there would have been no rainfall. With no rain there was no way to remove carbon dioxide from the atmosphere. However, tectonic activity would have continued, since it is driven by the warmth from the decay of natural radioactive materials deep underground. As crustal plates were subducted, rocks would have melted, releasing huge bubbles of carbon dioxide, which would have been expelled from volcanoes. Eventually, the carbon dioxide in the atmosphere would have built up enough to thaw the planet and allow the long-term carbon cycle to resume. This sequence of events occurred four times toward the end of the Proterozoic eon. The last of these four ice ages ended about 580 million years ago—just about the time when a wide variety of life forms evolved.
Hoffman, Schrag, and others have proposed that the explosion of complex animal life may have been stimulated by these severe ice ages. Fossil evidence indicates that before the deep freeze, Earth was populated with single-celled animals and simple plants. The extreme cold conditions would have wiped out all but some isolated colonies of organisms near deep ocean vents, or possibly on the surface of the ice, where they could absorb energy from the Sun. Evolution proceeds rapidly when a population is separated into isolated colonies. That is just what occurred during the “snowball Earth.” Each time the climate warmed, these colonies would have been able to multiply, move into areas that were just becoming habitable, and continue to evolve new species through the process of natural selection. Each new wave of extreme cold would have caused some species to become extinct. At the same time, some isolated colonies survived and evolved. By 580 million years ago, when Earth entered a long, relatively warm period, our planet was populated by many forms of multicellular plants and animals.
IV. Climate During the Carboniferous Period
(360 million to 286 million years ago)
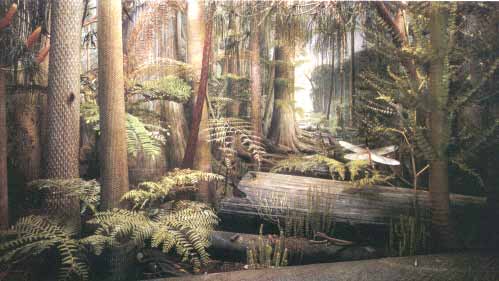
Courtesy of the Field Museum of Natural History, Chicago
During the Carboniferous period, life began to multiply on land, and the first woody trees grew into dense forests. These forests were an insect collector’s dream. At least 500 fossil insects from this period have been identified so far, including large cockroaches and mayflies.
The Carboniferous period began with a warm climate, favorable to a wide variety of life forms. Fossils from this period indicate large areas of forests and marshes with fernlike and seed-bearing plants. Feeding on these plants was a wide diversity of amphibians—animals that can live on land or in the water—and many species of insects, some of huge size. Fossils show dragonflies with three-foot wingspan! Although the Carboniferous is known as the Age of Amphibians, it is also the time when the first reptiles appeared.
Toward the end of the Carboniferous the climate was bitterly cold. Most theories hold that the cooling started with the shifting of continents. In the earlier Devonian period, the continents in the Southern Hemisphere had drifted and were joined together, forming a large land mass that scientists named Gondwana. As Gondwana drifted toward the South Pole, a glacier formed, and as the glacier became larger and larger, its gleaming white surface reflected sunlight into space—so Earth got even colder.
The large glacier at the South Pole tied up tremendous amounts of water, which caused the sea level to fall and expose huge areas that had previously been covered by shallow water. When naturally occurring acid rain fell on those areas, the rain dissolved large amounts of minerals, which mixed with carbon dioxide in the ocean. With more and more carbon trapped on the ocean bottom, the greenhouse effect decreased, and Earth cooled even more.
Most of the world’s coal, which is primarily carbon, formed during the Carboniferous period. That is how the period got its name. Geological evidence suggests that the coal-forming process started when the south polar ice cap melted, and lowlands and swamps on the continents were flooded. The rapidly rising water levels drowned forests and marshes. Sediments smothered the plants, which decayed partially and turned into peat. After thousands of years buried underground, the peat transformed into coal. When the ice cap built up again, sea levels dropped, and new forests grew, only to be flooded a few thousand years later.
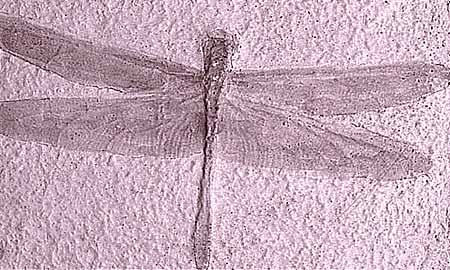
This beautifully preserved fossil of a dragonfly (photo at right) is from the Jurassic period. It is about 155 million years old. The wingspan of this specimen is about 15 centimeters (about 6 inches). The oldest fossils of this type of inset are form the Carboniferous period. These earlier insects had wingspans up to 75 cenimeters (30 inches).
V. Climate During the Cretaceous Period
(144 million to 65 million years ago)
The Mesozoic era is perhaps the best known geologic time span of Earth’s history. Even young children can name many of the dinosaurs that flourished during those times. The warmest part of the Mesozoic was the Cretaceous period. Almost all the continents were inhabited by a great variety of plants and animals. Huge palm trees grew at high latitudes, and coral reefs rich in sea life flourished.
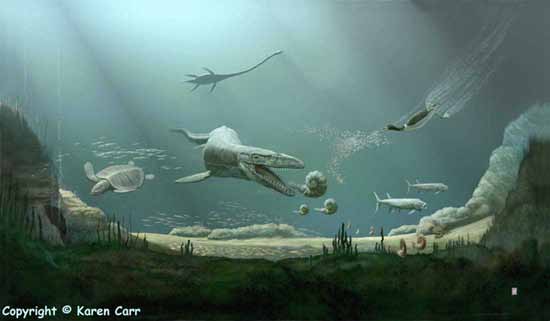
No one knows for certain why it became so hot during the Cretaceous period. However, sufficient evidence exists to enable paleoclimatologists—people who study ancient climate—to piece together a plausible explanation. No large continents were at the North or South Pole during the Cretaceous, so there were no polar ice caps. Pangaea had split into a northern and a southern continent separated by a large equatorial sea that extended nearly a third of the way around the world. The warm ocean currents from that sea were deflected northward and southward as they entered the huge world ocean that surrounded the continents. These currents carried warm tropical waters northward and southward, and acted like a huge heating system, warming the climate around the globe.
With a warmer climate and no significant polar ice caps, the sea levels rose worldwide and covered coastal areas with shallow water. Huge deposits of lava and ash provide evidence of extremely active volcanism during the Cretaceous period. Active volcanism meant that large amounts of carbon dioxide were injected into the atmosphere and the greenhouse effect increased. As a result of all these factors, the Cretaceous became the warmest period in Earth’s history.
What stopped the warming? In a warm climate there is rapid evaporation of ocean water and the formation of thick clouds. Clouds shade Earth and reflect more sunlight into space, which has the effect of cooling Earth. In addition, clouds increase the amount of rainfall. More rainfall means that more minerals would be washed out to sea where they would combine with carbon dioxide in the water to form carbonate rocks. With rock formation taking carbon dioxide out of the water, the ocean would absorb more carbon dioxide from the air. Thanks to the long-term carbon cycle, the greenhouse effect then lessens, and Earth becomes cooler.
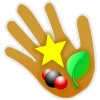
LC8.1. Investigation: Dissolving Rocks
Simulate the process of chemical weathering in the laboratory.
III. Conclusion
In the previous sections we saw how the slowly shifting continents and the ocean interacted with the atmosphere and brought about changes in Earth’s climate. As climate changed, so did life forms. Some forms adapted to new conditions, because the climate changes were usually slow. Through natural selection those forms that had the capacity to change and sufficient time adapted to the new conditions. But not all species survived. Even during periods when environmental change was slow, species would occasionally become extinct.
The fossil record also reveals that at certain times in Earth’s history events occurred that led to the massive extinction of huge numbers of species all at once. In fact, there have been at least five mass extinctions in Earth’s history. It has been estimated that of all the species that once lived on Earth, only one out of a thousand survives today. Many of the extinct species died during catastrophic events. The largest mass extinction was at the end of the Permian period. More than 90% of species living in the ocean and 65% of species that lived on land became extinct.
Another important extinction event occurred about 65 million years ago, when all of the dinosaurs and at least 50% of the plant and other animal life became extinct. What caused these catastrophes? In the next chapter, we’ll take a closer look at mass extinction events and the theories that have been developed to explain them.