AC6. Supernovae: Dramatic Change in Stars
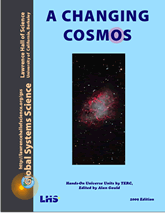
Chapter 6
I. What’s a Supernova?
We usually think of stars as very stable and constant things. Suppose you were out looking at the sky on a dark, starry night, and suddenly you saw a star that wasn’t there a moment earlier! You might think your eyes were playing tricks on you. You could check by taking a photograph of the region of the sky and comparing it to a photograph taken earlier of the same region. Normally, these photos would be roughly the same. Certain objects, such as planets, may have changed position relative to the background stars, and the brightness or size of the stars may appear different from night to night, but the arrangement of the stars relative to each other generally does not change over a period of nights, years or even centuries.
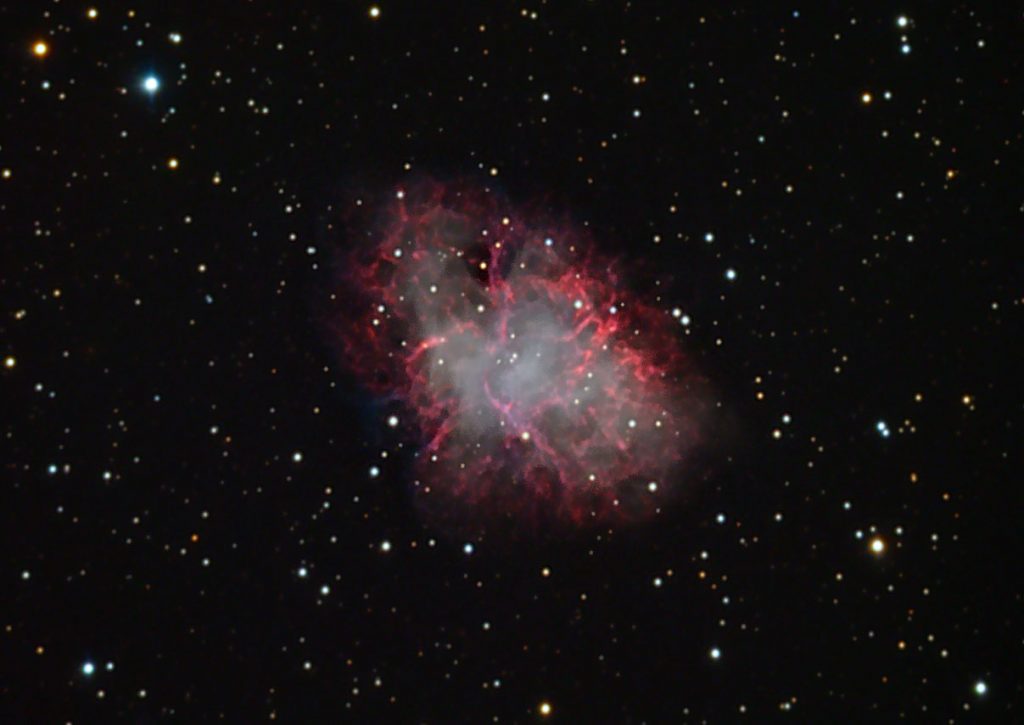
Image from Ewell Observatory, Belmont, CA.
A supernova could be an explanation for seeing a new star. The term comes from the Latin word “nova” meaning new, though ironically a supernova is actually the event associated with the death of a star—the unbelievably violent process that a very massive star undergoes when it dies. Many people think of supernovae as explosions, and in some cases this is true, but some stars implode rather than explode when they die. Astronomers have studied various types of supernovae and have created possible explanations for the processes that could cause such events. Certain types of supernovae can give important clues to the puzzle of the age, size and fate of our universe, as well as contribute to our evolving understanding of the structure of stars.
Although the study of supernovae is a very active field in astronomy, theories are constantly being challenged about the different types of supernovae and the stars that produce them. Currently more than sixty supernovae are discovered each year, but often times they are sighted after the maximum peak of the light curve so some of the scientific information is lost.
Astronomers have classified supernovae into two kinds, Type I and Type II, based on the amount of hydrogen observed in the material surrounding the explosion of the star. Type I have no observed hydrogen, leading astronomers to believe the outside layers were already shed. Hydrogen is observed from Type II supernovae, so they are generally believed to be explosions of higher mass stars. This theory is consistent with the fact that Type II supernovae are only found in spiral galaxies, and usually in the arms, where high-mass star formation is thought to be more prevalent, whereas Type I supernovae are found in both spiral and elliptical galaxies.
Most elements heavier than hydrogen and helium are predominantly created inside stars or in the process of the supernova explosion itself. These elements are ejected into space by supernovae and then reused to form new stars and planets such as the Earth. The atoms that make up almost every substance that you deal with everyday, including the chair you are sitting in, the food you eat, and even your body itself, were once inside a star. You are made up of star matter.
II. More Photometry Terms
The search for supernovae entails using photometry again, with the image processor photometry tool. In addition to the terms we used in Chapter 4 (Intensity Value, Apparent Brightness, Luminosity, Apparent Magnitude, and Absolute Magnitude), you need to know two more:
Reference Star
A star whose apparent brightness and luminosity does not change from one night to the next. The apparent brightness value of the star, however, is typically not known.
Standard Star
A steady star is like a reference star but with a known, agreed upon value of apparent brightness.
III. Searching for Supernovae
Both amateur and professional astronomers actively search for supernovae, and they are being discovered more and more frequently. Using the same basic strategy, they look for the appearance of a new star. Supernova SN1994I was the first supernova discovered by Hands-On Universe students at Oil City High School in Pennsylvania. The discovery was based on images taken for them via the Internet with an HOU telescope. Supernova search is an area of research for HOU students. How does it work?
To get ready for the Investigation “Finding a Supernova” you’ll need to know something about photometry—the measurement of light. Sometimes a supernova is so bright it can be spotted by eye by just looking at two images taken at different times. In fact, there is an amateur astronomer in Australia, a minister named Bob Evans, who can look at a galaxy and compare it with his memory of this same galaxy at a previous date. Very impressive. For most of us, this technique is beyond our capabilities.
But a quite reliable strategy is to compare images of the same object using image processing tools. Images of a galaxy before a supernova have occured are called reference images. The first steps in this strategy (A through C) are to be sure that all images line up perfectly and brightness deviations be accounted for:
A. Alignment
the telescope does not perfectly line up on the object the same each night, so images appear off-center, or shifted with respect to one another.
Corrected with shifting, also known as aligning
(adding a correction displacement x-value and y-value to each pixel’s x and y coordinate, respectively).
B. Sky background light
can vary significantly from image to image due to ground lights and moonlight.
Corrected with Sky background subtraction.
(subtracting a constant intensity value a correction factor from all pixels).
C. Exposure time and observing conditions
such as cloudiness or haze, can change from night to night, so the apparent brightness of all the stars may seem dimmer or brighter. Light we are receiving from stars has traveled vast distances. Amazingly, the light remains virtually unaffected by the first 99.999999999999% or so of its journey. But the last tiny leg of the trip through the Earth’s atmosphere can reduce the light drastically and star brightness can differ from one observation to the next.
These irregularities are corrected with a process called
Normalization (multiplying all pixel intensity values by a correction factor).
Only after the images are properly shifted, sky adjusted, and normalized,
are we’re ready for the final step:
D. Compare the images
and identify any new light sources not in the reference image(s).
This can be done one of two ways:i. Subtraction: you can subtract one image from the other to examine the difference. If a significant variation has occurred, such as a supernova, it will be apparent in the subtracted image.
ii. Blinking: switching the image display alternately from one image to another can make any object that has change nearly “jump out at you.”
The table below gives more explanation about what happens in steps A, B, and C.
A. Align Files by Shifting
In order to align two images so they are ready for subtraction you need to use the Axes (Centroid) tool in Data Tools and the Shift option in Manipulation. What happens when you shift a file? At right is data from a small section of an image file with intensity values given for the pixel locations to illustrate the result when the image processor shifts a file “to the right by 2”
In this case as the image was shifted to the right, the new columns created on the left were filled in with zeroes. There is an option in the software to select a “fill value” so that you can have new columns or rows filled in with a value that matches the background sky.
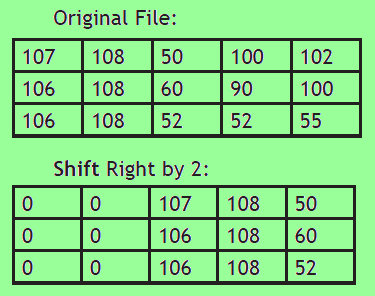
B. Subtract Sky Background
Differences in brightness may be due to changes in the background light level, called “sky”, such as the amount of Moon light.
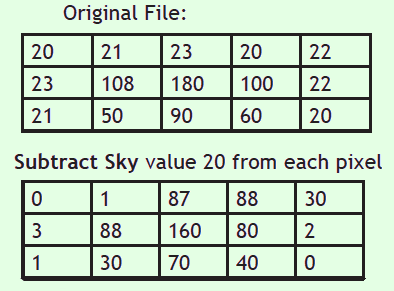
C. Normalize Brightness
To correct for differences due to change in exposure time, compare the intensity values of a reference star (a star that is known to be of constant brightness) in each image. Find the “reference ratio” of intensity values for the reference star in one image divided by the intensity values for the same reference star in the second image. Then multiply the second image by the reference ratio. Reference ratio is often referred to as normalization ratio.
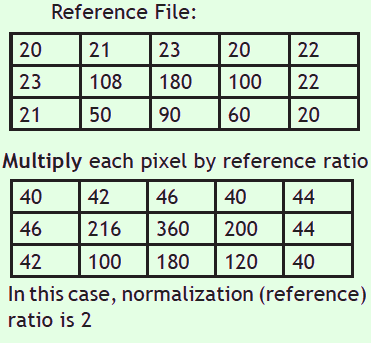
Note:
- Steps A and B can be reversed, but normalization is best to do last, just before final subtraction/ comparison of the two images.
- Although both steps B and D are subtractions,
in B the same intensity value (sky background) is subtracted from every image pixel, but in D the intensity value of each pixel in a reference image is subtracted from the corresponding pixel in the new image.
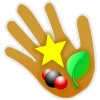
AC6.1. Investigation: Finding Supernovae
Here are a series of exercises on finding supernovae, starting with simple specially prepared images and progressing to real life image analyses.
IV. Science of Supernovae
Type I Supernovae
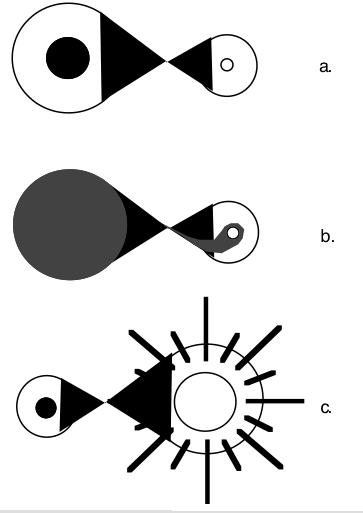
Figure 1
a) A star and its companion white dwarf within their Roche lobes.
b) The star bloats into a red giant and its outer layers overflow the Roche lobes. The overflowing matter falls onto the surface of the white dwarf.
c) The white dwarf implodes and ignites fusion causing a bright flash called a Type I supernova.
Most stars in the Universe are found in multiple star systems, meaning that two or more stars are in a gravitational orbit around a common center point. When these stars are very close together the material from one star can spill over onto another star, greatly effecting the evolutionary process of each star. Current theories suggest that Type I supernovae occur in binary systems containing a white dwarf and a massive star.
A white dwarf is the very compact remnant of a low mass star that has burned up all the hydrogen and helium in its core leaving a very dense remnant of mostly carbon. The outer layers of unburned hydrogen were blown off during a burst of helium fusion that created a planetary nebula around the white dwarf. A white dwarf is always less than 1.4 times the mass of the Sun. Any additional mass will cause the white dwarf to collapse and create a different type of remnant called a neutron star.
When a white dwarf is part of a binary, mass can be exchanged between the white dwarf and its companion. Each star has an imaginary shell around it within which all matter is gravitationally bound to that star. Astronomers refer to these regions as the Roche lobes for the binary system (see figure 1). As the companion star evolves, its radius will expand due to thermal pressure. This may cause some of its outer material to overflow its Roche lobe and fall onto the white dwarf. The white dwarf gains more and more mass by this method until it reaches the critical threshold of 1.4 times the mass of the Sun, where it can no longer support itself. In a violent implosion, called a Type Ia supernova, the white dwarf succumbs to the increased pressure and, in turn, heats up to the point where it can burn fuel again. This time the fuel is carbon. The ignition of the fuel results in a tremendously bright flash, which then fades over a period of days or weeks. Discoveries of these supernova have been made out to the edge of the visible universe.
The critical threshold of 1.4 times the mass of the Sun is the same for all white dwarfs. This means that no matter what the mass or temperature was for the original star, it will implode with the same amount of fuel left to burn. Since the mechanism for ignition is the same and the amount of fuel is the same, it follows that the luminosity resulting from the rapid ignition is the same for all white dwarfs undergoing a Type Ia supernova. Astronomers call such an object a “standard candle”, meaning that its luminosity is known so we can use it as a point of reference from which to compare other objects. We can observe the apparent brightness of the supernova as seen from earth, and knowing its absolute brightness as a standard candle, we can then determine its distance away from us.
Type I supernovae are often categorized as Type Ia, Type Ib, or Type Ic supernovae. The different letters refer to differences in the specific elements detected after the explosion and the rate at which its brightness fades. Theories that attempt to explain the differences among the various categories of Type I supernovae focus on the specific mass of the original star. It is thought that a Type Ib or Ic supernova may be caused by the remnant of a very high mass star, such as a neutron star that is part of a binary system.
Type II Supernovae
High mass stars undergo even more violent explosions called Type II supernovae (see Figure 2). High mass stars achieve much higher temperatures inside so they are able to burn heavier elements than low mass stars. A very dense core of iron builds up within the center of the star as a result of the burning, with the lighter elements in the surrounding layers.
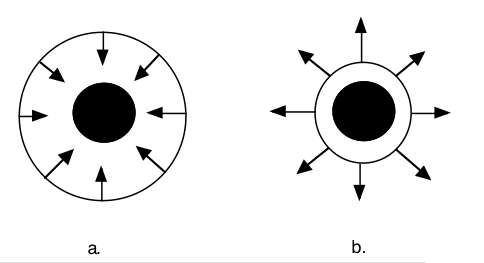
Figure 2
a) In a Type II supernova the outer layers of a high mass star come crashing in and
b) bounce off the dense core sending a shock wave outward.
This configuration is sometimes referred to as an onionskin model because of the spherical shells of various elements.
Through energy-producing nuclear fusion, only elements as heavy as iron can be produced. Any nuclear reactions producing heavier elements require an input of surplus energy. Therefore the star only continues to burn fuel until iron is produced in the core and then fusion stops. After the fuel runs out, the core cools to the point where the gravitational pressure causes the star to come crashing in on itself. The implosion is so strong that the outer layers of the star crash into the hard iron core and bounce back out with tremendous energy. This is called a shock wave. The shock wave ignites the material in the outer layers of the star and the result is a sudden explosion that can be one billion times as bright as the original star. The remnant of the core of a Type II supernova will either be a neutron star or a black hole, depending on the original mass of the star.
The intense brightness of a Type II supernova is caused by the burning of the lighter elements that are in the outer layers of the star. This material is thrust outward by the explosion, creating an expanding bright nebula or halo that can remain visible for thousands of years. The explosion releases such tremendous amounts of energy that the surplus energy required for nuclear fusion of elements heavier than iron is available. In fact, it is believed that supernova explosions may be responsible for the creation of all material heavier than iron or at least for providing “seed” iron elements that are the fuel for further nuclear and chemical evolution. This includes elements such as lead, zinc, gold, and silver.
Neutron Stars and Black Holes
The two different types of remnant cores from supernovae, neutron stars and black holes, can be understood by trying to imagine unimaginable densities of materials. In a neutron star, the density of material is so great that the pressure on each atom exceeds the ability of the atom to maintain its structure of electrons surrounding a nucleus with protons and neutrons. The negatively charged electrons are forced right into the nucleus where they combine with the positively charged protons, producing a material made of nothing but neutrons.
An entire neutron star may have a diameter of only between 10 and 20 km. With the entire mass of the star compressed into that small of a space, the density is practically inconceivable—about 1017 kg/m3 . Compare that with the average density of Earth, about 5,000 kg/m3. Astronomers enjoy saying that a teaspoon of neutron star material would weigh around a billion tons.
The rotation rate of the object changing from an ordinary star into a neutron star increases dramatically, like a figure skater doing an spin and bringing his or her arms in to increase the spin rate. Only with a neutron star, the amount of compression of material can result in spin rates of thousands of times per second. Neutron stars emit radiation that pulses at those high rates, and such objects have been dubbed “pulsars.”
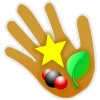
AC6.1b. Investigation: Discovery of Pulsars
In 1967 a Ph.D. student at Cambridge University assisting the astronomer Anthony Hewish made a discovery that altered our perception of the universe. That Ph.D. student was Jocelyn Bell Burnell. She discovered pulsars — compact, spinning celestial objects that give off beams of radiation, like cosmic lighthouses. But the world wasn’t ready to accept that a breakthrough in astrophysics could come from a young woman.
Watch this movie about Jocelyn Bell Burnell and discuss your thoughts about it with friends & family.
If understanding what a neutron star is involves unimaginable densities, that’s nothing compared with the density of a black hole. The actual diameter may not be a lot bigger than that of a neutron star, but a black hole has many times more mass packed in that space. One way of thinking about a strange characteristic of a black hole is to think about the idea of escape velocity. On Earth if a rocket ship achieves a speed of a little over 11 km/sec, it can escape Earth altogether. So the escape velocity from Earth is about 11 m/sec. In general, the more massive and dense the planet, the greater its escape velocity. For a black hole, the mass and density is so great that the escape velocity is greater than the speed of light (3×108 m/sec). Light can be in orbit around a black hole, creating a “photon sphere.”
You might ask, “If light can’t escape a black hole, how could you possibly see it?” You can’t see it directly. Like many things in astronomy, they can be detected by how they affect surrounding objects. The first black hole that was discovered was in a binary star system called Cygnus X-1 where there is a “normal” star and a black hole orbiting each other. It is an intense source of X-rays, which is an indicator that the black hole is present.
V. Variable Stars
6.20. The six graphs shown below are graphs of data (light curves) from different stars.
What is happening at each of these stars that is causing its brightness to change?
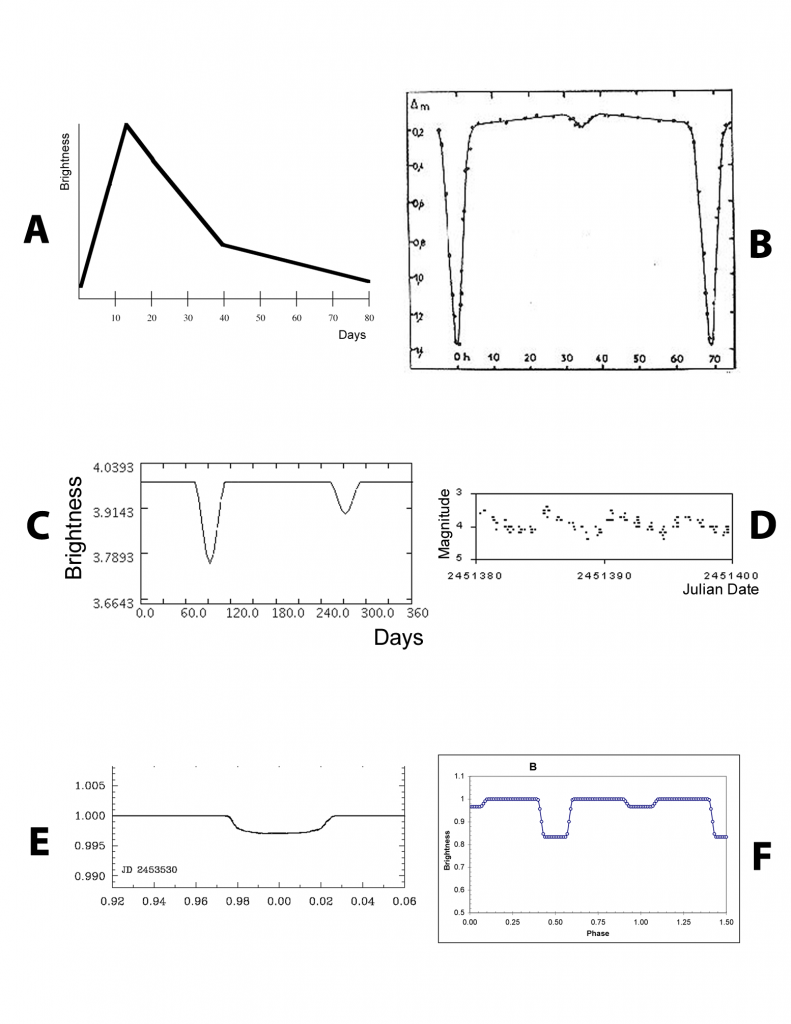
What would happen with two stars orbiting each other (binary star)?
What would cause brightness to change? What factors would affect the shape of the curve?
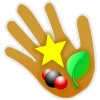
AC6.2. Investigation:
Eclipsing Binary Stars
Investigate eclipsing binary star systems using observations from the American Association of Variable Star Observers.
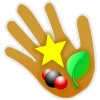
AC6.3. Investigation:
Discovery of Gravitational Waves
2016-02-11. Gravitational waves, Einstein’s ripples in spacetime, spotted for first time. By Adrian Cho, Science. Excerpt: …two massive black holes—the ultrastrong gravitational fields left behind by gigantic stars that collapsed to infinitesimal points—slowly drew together… spiraled ever closer, until, about 1.3 billion years ago, they whirled about each other at half the speed of light and finally merged. The collision sent a shudder through the universe: ripples in the fabric of space and time called gravitational waves. Five months ago, they washed past Earth. And, for the first time, physicists detected the waves, fulfilling a 4-decade quest and opening new eyes on the heavens. The discovery marks a triumph for the 1000 physicists with the Laser Interferometer Gravitational-Wave Observatory (LIGO), a pair of gigantic instruments in Hanford, Washington, and Livingston, Louisiana. …Albert Einstein predicted the existence of gravitational waves 100 years ago, but directly detecting them required mind-boggling technological prowess …[sensing] a wave that stretched space by one part in 1021, making the entire Earth expand and contract by 1/100,000 of a nanometer, about the width of an atomic nucleus….. Read the entire Science Magazine article at http://www.sciencemag.org/news/2016/02/gravitational-waves-einstein-s-ripples-spacetime-spotted-first-time — see also New York Times video and article, MIT News story, and Remembering Joseph Weber, the controversial pioneer of gravitational waves.
Also watch a recorded seminar given by physicist Daniel Holz to a group at Lawrence Berkeley National Laboratory. Then write your own views on what this discovery was and what its significance is.
See Staying current for this chapter.