LB4. The Puzzle of Inheritance
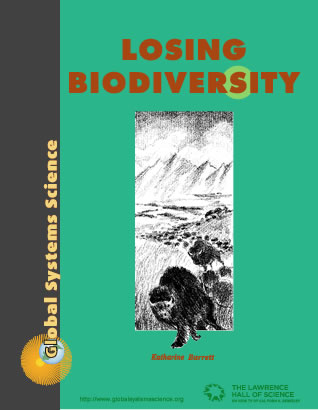
Chapter 4
How did millions of different kinds of organisms evolve?
Question 4.1
Looking for human variation. Choose an easily visible trait like shape of face, nose, or mouth, and make a tally of all the different shapes you see on a TV news program that zooms in on people around the world.
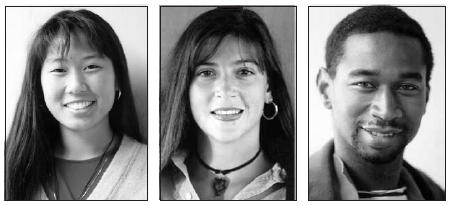
Darwin hypothesized that living organisms were descended from a common ancestor. He and Wallace proposed that natural selection—operating on generation after generation of offspring—eventually resulted in the incredible variety of life forms we see around us today. They didn’t have powerful microscopes and modern methods of estimating the age of the Earth and its fossils. They assumed that early life was simple in form and arose a very long time ago.
Evidence suggests that the Earth cooled enough to form solid continents and oceans about four billion years ago. By 3.6 billion years ago, and perhaps even earlier, the first forms of life began to multiply in the silt at the bottom of our planet’s shallow seas. Paleontologists (scientists who study fossils) have discovered evidence of ancient bacteria in the very oldest rocks.
Bacteria are tiny organisms, so tiny that 10,000 can fit on the head of a pin. They can reproduce quickly, some as often as once every twenty minutes! In most cases, bacterial reproduction results in new bacteria that are exactly alike. They are the same size and shape, and break down the same kinds of matter in the same ways. In some cases, however, bacteria produce offspring that have a new trait or lack a trait from the parents.
Scientists use the term “mutation” to refer to a new characteristic that appears in an organism and can be inherited by its offspring. Mutations are rare, and most organisms with a mutation die or fail to reproduce. Once in awhile however, an organism with a mutation turns out to be better adapted to the environment and survives to produce offspring with the different set of traits. It was this normal process of passing on traits from parents to offspring, and occasional mutation, that made evolution possible. This process led from the first bacterium to the wide diversity of life that we see today.
Up until the late 20th century, the most common classification scheme for life was five kingdoms. This diagram shows relationships among life forms that descended from ancient bacteria (Kingdom Monera) through the processes of mutation, inheritance, and natural selection. The kingdoms classification has been widely replaced by a scheme with three domains that will be shown later in this chapter.
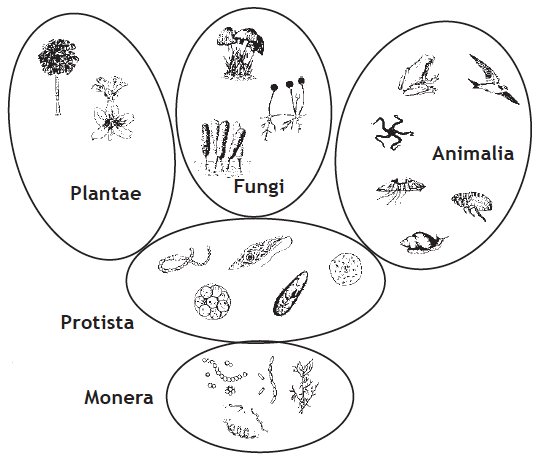
Darwin was able to explain why some mutations survived and others didn’t, but he could not explain how organisms passed on traits such as eye color to their offspring, or how mutations occurred. He knew that a process of inheritance must exist, but he didn’t know how it worked. The understanding of inheritance is now the focus of an entire field of science called genetics. Because genetics is so important to understanding the problem of losing biodiversity, we will devote this entire chapter to it. By the end of the chapter you should understand the answers to several puzzles that Darwin was unable to solve:
- How do organisms pass on their traits to their offspring?
- Why do offspring have only some of the traits of their parents but not others?
- Where is inheritance located?
- What causes mutations?
- How is inheritance related to biodiversity?
I. How Do Organisms Pass on Their Traits?
The earliest inheritance research was done by Father Gregor Mendel, who experimented with plants. From 1853 to 1864, he grew pea plants in a monastery garden in Moravia, which is now part of the Czech Republic. Pea plants show a variety of different traits, such as: tall or short plants; wrinkled or smooth seeds; white or red flowers. Mendel chose to study traits he suspected could be passed on in the seeds to the next generation of pea plants.
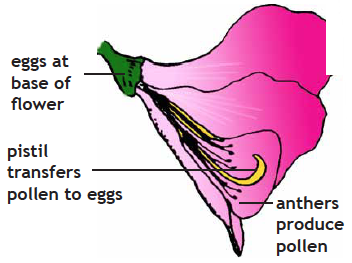
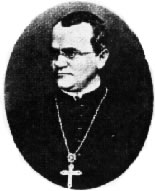
The flower of the pea plant has both male and female structures. Pollen from the male anthers travels down the female pistil to fertilize the eggs at the base of the flower. Each fertilized egg may then develop into a seed. To crossbreed flowers from pure strains, Mendel placed the pollen from red flowers onto the pistils of white flowers; He also put the pollen of white flowers onto the pistils of red flowers.
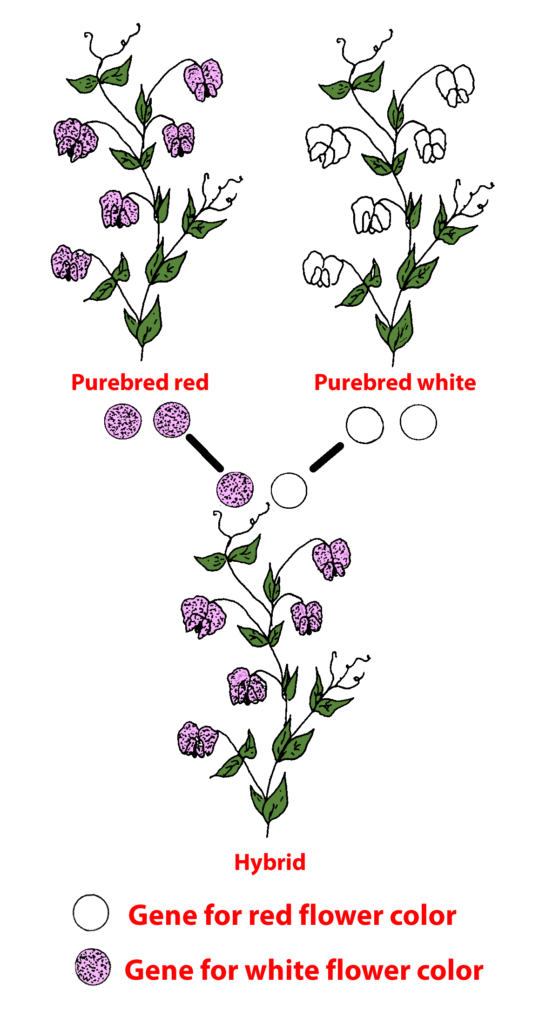
Perhaps he thought the traits would mix, producing plants with pink flowers, or that half the offspring would have white flowers and the other half, red flowers. Instead, he found that all of the crossbred plants, or hybrids as he called them, had red flowers. Why weren’t there any white flowered plants? Where was the trait for white flowers? Had it been lost?
Mendel discovered that the trait for white flowers was not lost. When he crossbred the red hybrid plants, he found that about one out of every four offspring had white flowers! When he crossed the white flowered plants in the next generation, they turned out to be “pure.” That is, they never produced red flowers.
Mendel concluded that traits like flower color are determined by individual units, which he called “factors.” He proposed that these factors are passed on in the seeds of the plants, and that each trait in an offspring is determined by a pair of factors—one factor from the pollen and one factor from the egg. Today we call these factors “genes.”
Mendel’s work revealed that some genes, like the red-flower gene, could hide the second gene. He called these genes that are always visible “dominant.” He called the genes that are hidden by dominant genes “recessive,” as in the case of the recessive gene for white flowers.
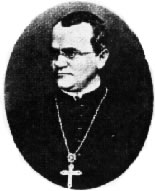
See article in Science: Massive pea study solves last genetic riddles of famed friar.
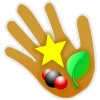
LB4.1. Investigation: Hybrid Cross Coin Toss
You can simulate Mendel’s hybrid crosses by flipping two coins at the same time and observing how the pair lands. Each side of a coin represents a “coin gene” with heads the dominant trait.
The box shown here, called a Punnett Square, has the possible combinations of the “coin genes” and their probabilities of landing with two heads, two tails, or one of each.
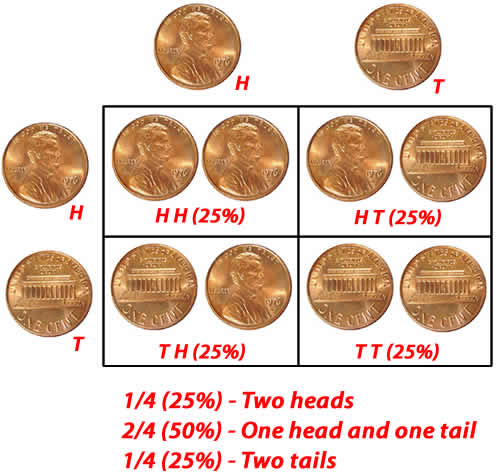
- Toss a pair of coins 20 times.
- Keep a tally of how they land.
- Compare your results with the mathematical probabilities shown in the Punnett square.
- How do you think your results might change if you toss the coins 100 times?
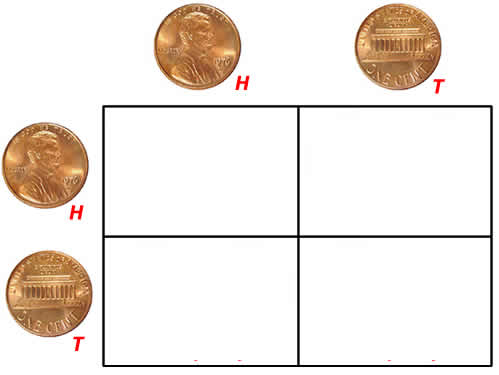
II. Where Is Inheritance Located? Inside Cells
With the development of better microscopes, scientists observed that plants and animals of all sizes and shapes are made up of tiny units called “cells.” The cells of different organisms differ in many ways, but all cells appear to have some basic features in common, including the ability to divide and form two new cells.
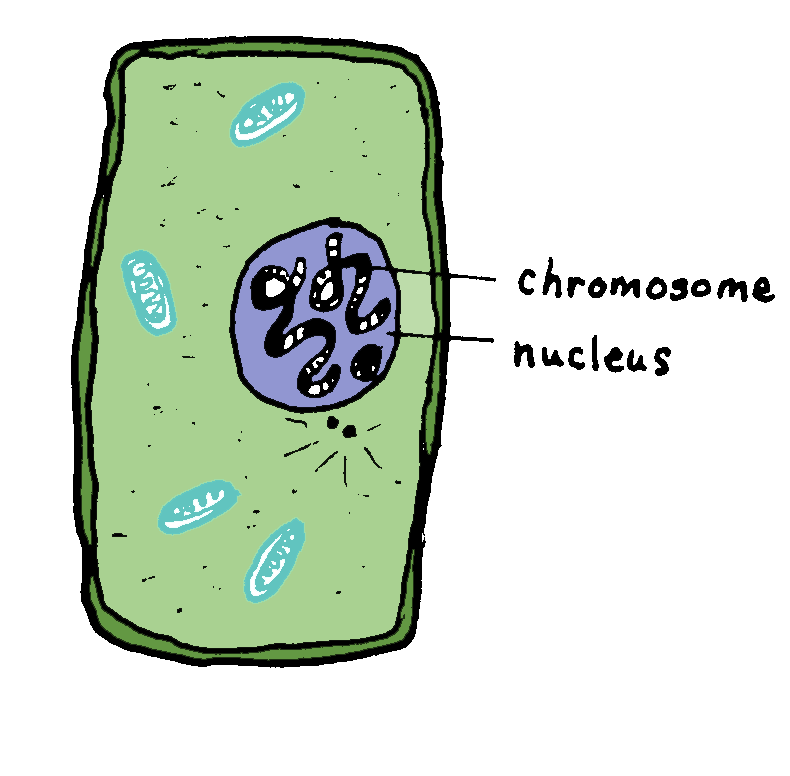
Each of us began life as an egg cell from our mother, fertilized by a sperm cell from our father. The fertilized egg divided and formed two cells. Each of those cells divided to form four cells. The growth and divisions of the cells continued eventually forming all of the muscle cells, nerve cells, bone and body cells that make us a human.
Careful comparisons of cells in different stages of growth reveal microscopic structures that are connected to inheritance. Scientists found that the cells of plants and animals have a control center called the “nucleus.” The nucleus is enclosed by a membrane, and contains thread-like structures, called “chromosomes.”
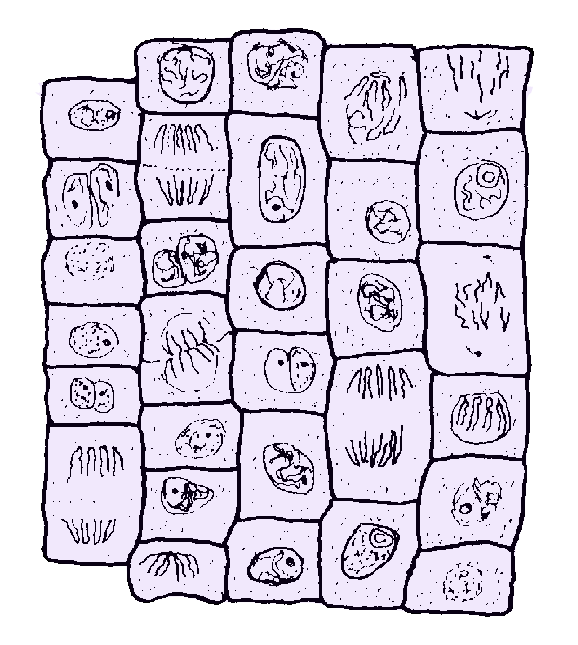
During the life of the cell, the chromosomes go through a regular series of changes leading up to division of the nucleus and, in most cases, the formation of two new cells.
Chromosomes occur in pairs, and scientists inferred that the chromosomes contain the paired genes predicted by Mendel. Studies revealed that each parent contributes one of the paired chromosomes. As an organism’s cells divide, exact copies of the chromosome pairs are made for each new body cell. Different species of organisms often have different numbers of paired chromosomes. Humans, for example, have 23 pairs of chromosomes in most cells of the body.
III. Cells Divide to Form New Cells
This diagram shows the process of “mitosis” in an animal cell. The nucleus divides to form two identical new nuclei, each in a new cell.
Mitosis in an animal cell
Before mitosis begins, the chromosomes in the nucleus are not very visible (A), however, once cell division begins, the chromosomes condense and look like long, thin “X’s.”
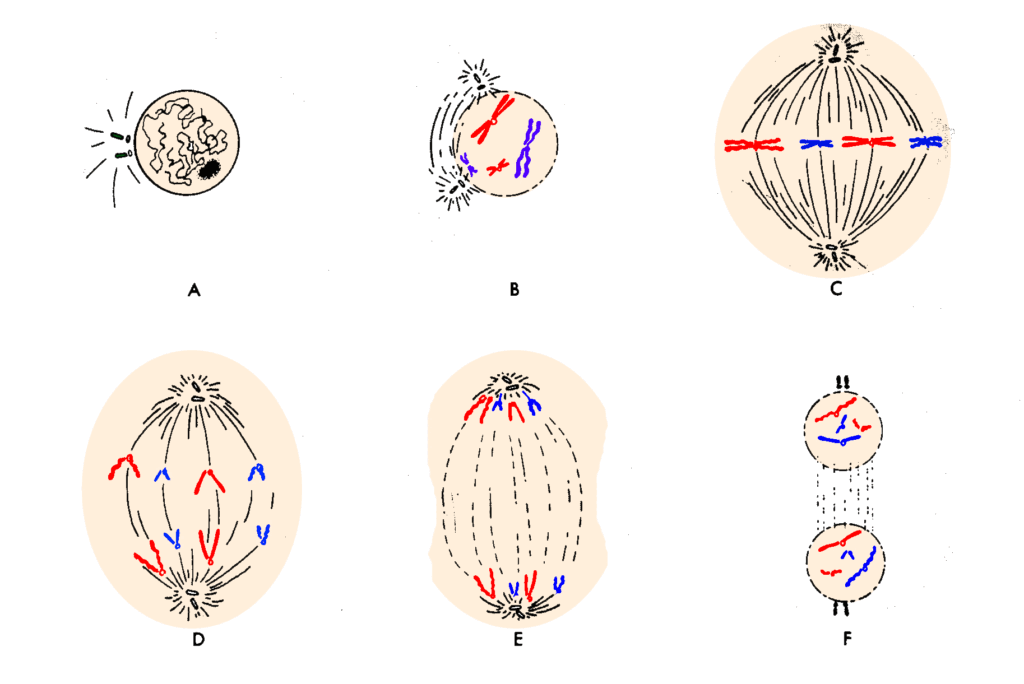
This first step of mitosis is shown in cell B. It is during this step that the chromosomes replicate so that now there are two sets of chromosomes.
In step C, the chromosomes move towards the center of the cell. The chromosomes then separate from each other during (D) and move to opposite ends of the cell (E). During the final stages of mitosis, the nuclear membrane pinches together to form two separate nuclei. Also the cell membrane pinches in to complete the formation of two new cells. It’s important to remember that the cells resulting from mitosis are identical.
IV. Why Do Offspring Have Only Some Traits of Their Parents?
Biologists also figured out the steps of another type of cell division known as “meiosis” that forms the male and female sex cells. Each sex cell contains only one of each kind of chromosome from the parent. The diagram below shows a plant reproductive cell (A) dividing to produce 4 pollen sex cells (I).
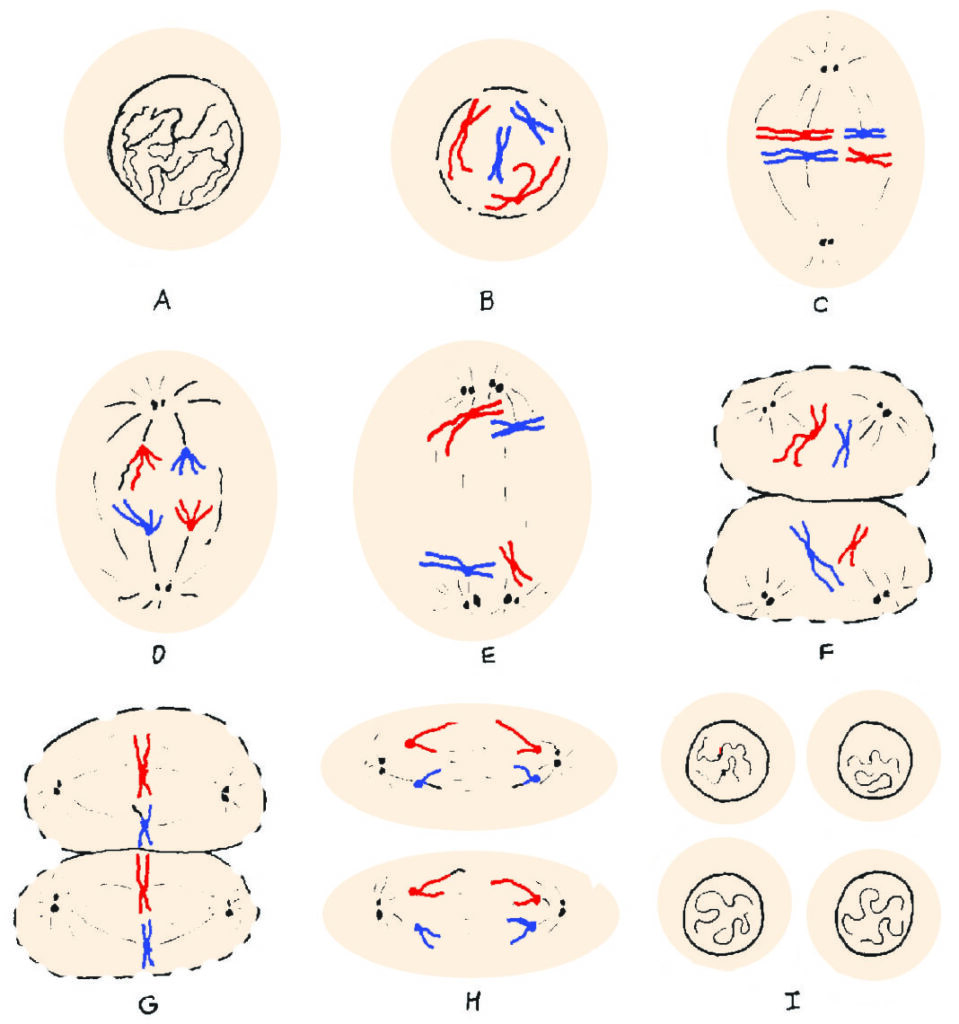
When a reproductive cell is ready to divide, the thread-like chromosomes duplicate themselves and become coiled thereby appearing shorter and fatter. The reproductive cell A starts with 2 pairs of chromosomes. Lets assume the long pair of chromosomes carries the traits for height, and the short pair carries the traits for color.
In step B, both long chromosomes and both short chromosomes have made copies of themselves. The copies are still attached to the original chromosomes. In steps C, D, and E, the chromosomes and their duplicates pair up, then get pulled into separate cells during division. In step F, each new cell has one long chromosome and its duplicate and one short chromosome and its duplicate.
Division occurs again in G and H, so that each new cell in I contains one long and one short chromosome. This process of reproductive cell division is called meiosis. Each new sex cell contains only one of each kind of chromosome from the parent. When sex cells from different individuals join, the new organism will have two of each kind of chromosome (2 long chromosomes with the genes for height, and 2 short chromosomes with the genes for color). The offspring that develops will have copies of half of each parent’s chromosomes. The evolution of the process of meiosis increased variation in populations by bringing together new combinations of genes in the offspring.
V. What Causes Mutations?
There was a great flurry of interest in heredity in the early 1900’s. Improved technology and the use of mathematical models made it possible to detect errors in the duplication of chromosomes. Sometimes strands of the chromosomes get tangled, break, and fragments exchange places. It turns out that this accidental exchange of genes, called “crossing over,” is relatively common, and is an important source of variation in organisms.
1. Each chromosome is attached to a copy of itself. Some “legs” cross over.
2. Pieces that are “crossed over trade places.
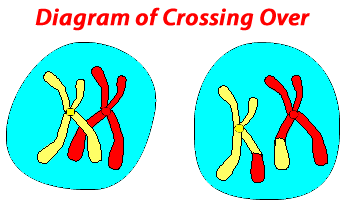
VI. Mapping the Location of Genes
In 1933, Thomas Morgan won the Nobel Prize in medicine for his Theory of the Gene, which he developed over a period of twenty years of studying fruit flies. These flies have just four pairs of chromosomes but the ones in the salivary glands are huge and have bands or stripes along their length. Morgan proposed that control centers or “genes” for the various characteristics of the flies, such as wing shape and eye color are located on the chromosomes. He kept careful records, and with his knowledge of crossing over and use of mathematics, was able to map the location of many genes on the chromosomes of fruit flies.
Scientists like Morgan, observed changes in the pattern of bands along the chromosomes and related these to specific changes in the characteristics of an organism. They searched for causes of these unusual differences, and found through experiments with ultraviolet radiation, radioactive materials, and chemicals that the chromosomes could be damaged easily resulting in errors or “mutations.”
Dr. Barbara McClintock showed that there were other ways that chromosomes can change. Studying the genetics of corn in the 1950’s, she discovered that genes move or “jump” from one spot to another on the chromosomes. Her work has helped us understand the evolution of human diseases in which “jumping genes” enable bacteria to mutate and develop resistance to antibiotics.
Organisms normally have pairs of each kind of chromosome, but scientists observed that plants sometimes have more than one pair of each kind of chromosome. They observed that additional sets of chromosomes can be added by chromosome mutation. Organisms with three or more sets of each kind of chromosome are called “polyploid.” For example, there are species of roses with 14, 21, 28, 35, 42, and 56 chromosomes. Crop plants such as wheat, potatoes and bananas are also polyploid. If a polyploid is capable of producing fertile offspring, but can’t interbreed with its parent species, a new species may be produced.
Mutations are a double-edged sword. They provide a population of organisms with new traits for surviving more difficult conditions. These traits can potentially be beneficial. However, most mutations are harmful, resulting in death of the offspring or failure to reproduce. These discoveries of genes, chromosomes and mutations provided more pieces of the puzzle to explain how traits are inherited and how occasional mutations occur.
VII. DNA: The Final Piece of the Puzzle
While the study of genetics was making major strides forward through crossbreeding studies, biochemists were identifying the molecular building blocks of cells. They found that the long strands of chromosomes were giant molecules of deoxyribonucleic acid, called DNA for short. It turned out that DNA was made up of three kinds of smaller molecules: a sugar, a phosphate group, and a nitrogen base. How these building blocks could carry and copy traits was still a mystery in 1951 when James Watson and Francis Crick decided to work together to identify the structure of DNA.
Watson and Crick experimented with models to determine how the building blocks are arranged— a sugar, joined to a phosphate group, and a nitrogen base form what is called a “nucleotide.” The diagram below shows how they arranged the nucleotides into a ladder-like structure. The rungs of the ladder are pairs of nitrogen bases that always pair in the same way. For example, adenine and thymine pair together, and cytosine and guanine pair together. Weak chemical forces, called hydrogen bonds, join the nitrogen bases, but can break easily when the DNA molecule separates into two complementary strands.
Diagram of DNA
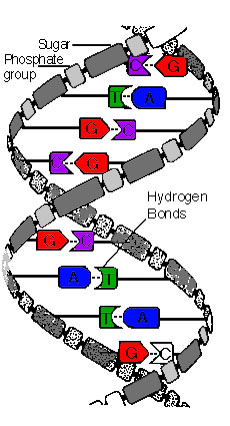
The nitrogen bases on a DNA strand provide a code for the various proteins every cell needs to function. A single DNA molecule can code for thousands of proteins. We now know that, in general, a gene is a part of a DNA molecule that codes for a particular protein. A small mutation in the DNA code causes changes in proteins. Some of these changes result in genetic diseases such as cystic fibrosis, Tay-Sachs, and sickle cell anemia. The diagram below compares the DNA genetic codes for normal and sickle-cell hemoglobin.
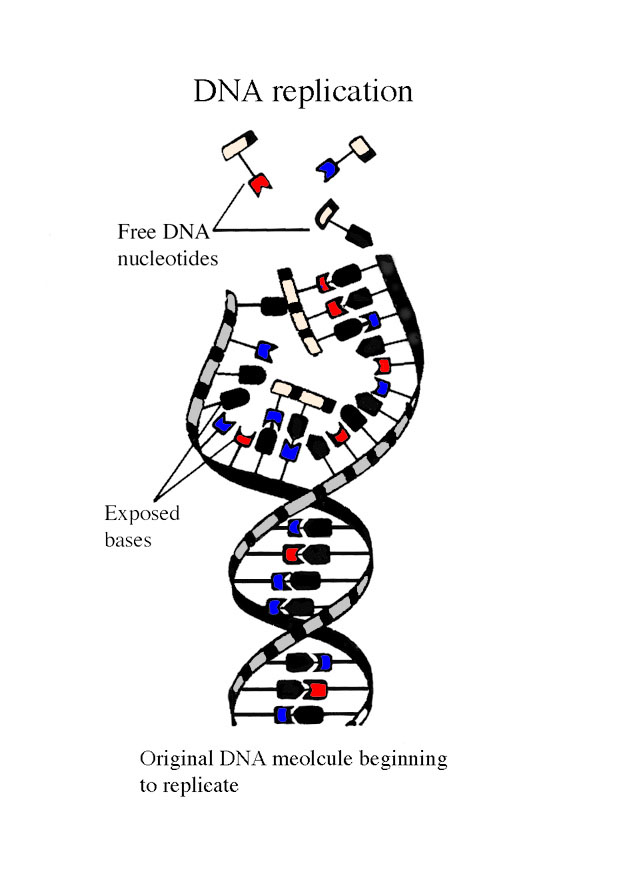
DNA replicates or copies itself during cell division. This copying process begins when part of the DNA molecule unwinds. The hydrogen bonds in that part of the molecule break and the two complementary strands separate, exposing two rows of nitrogen bases. Free DNA nucleotides that are present in the nucleus of the cell begin to match up and form hydrogen bonds with the exposed bases on each strand. Each strand of the original DNA molecule acts as a pattern, or template, to form the new DNA strand.
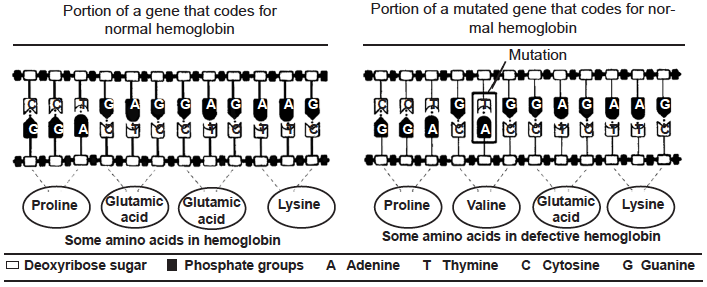
Remember the diagram of kingdoms of life that was near the beginning of this chapter? With understanding of genes and DNA, the newer classification with three large domains of life makes sense in terms of genetic similarities between the various subbranches with the domains.
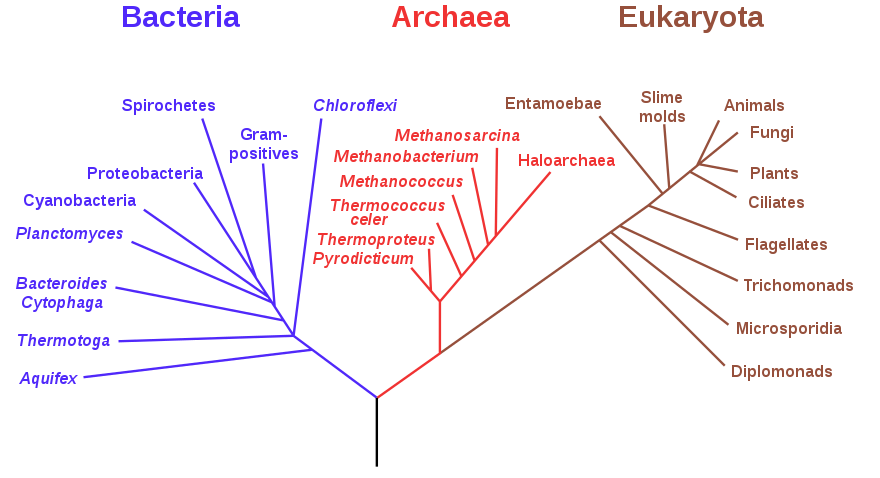
With increasing focus on comparisons of genes at the molecular level as a more important factor in classification schemes, genetic similarity is now viewed as a better criterion than outward appearances and behavior. The kingdoms categorization is now commonly replaced by three large divisions called domains. Carl Woese divided the prokaryotes (previously classified as the Kingdom Monera) into two groups, called Eubacteria and Archaebacteria because there is as much genetic difference between these two groups as between either of them and all eukaryotes.

VIII. Natural Selection for Sickle-Cell
Malaria is caused by a tiny protozoan that gets into the blood stream when a person is bitten by a mosquito. The protozoa burrow into the blood cells and multiply. When the blood cells burst, thousands of protozoa enter new blood cells. African people have a long history of exposure to malaria, which is one of the major causes of death in tropical areas of the world.
Perhaps as long as a million years ago, a mutation occurred in an African person’s genes that caused the hemoglobin protein in the red blood cells to develop in a crescent or “sickle-cell” shape instead of the normal round shape. Children who inherited the abnormal sickle-cell gene from both parents died during infancy from blood disorders such as anemia and blood clots. Today thousands of babies in Africa die each year from sickle-cell anemia. If the mutated gene was killing individuals, why didn’t it disappear from the African population?\
Normal red blood cells
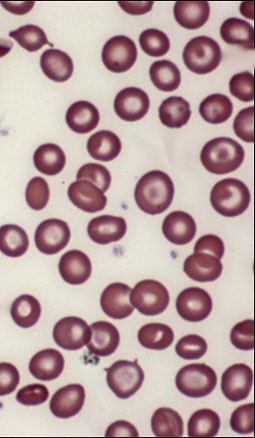
The answer to this question is twofold.
- The mutation was a recessive trait, meaning that it was hidden if the person also had the gene for normal blood cells.
- Africans who carried one recessive sickle-cell gene and one normal gene were more resistant to malaria and seldom died from the malaria parasite. These individuals passed the recessive gene on to their children who were also more resistant to malaria if they had one sickle-cell gene and one normal gene.
Sickle cell anemia cells
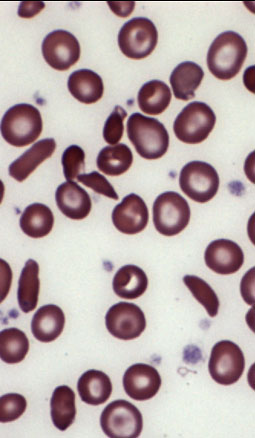
Africans who had two normal genes were more likely to die from malaria than people who carried the recessive trait. Apparently, having one normal gene and one sickle-cell gene provided an advantage in a place where malaria was common.
The total number of genes in a population is called the “gene pool.” Over thousands of years the frequency of the sickle-cell trait increased in the African “gene pool” due to natural selection of individuals who were better adapted to survive in an environment where malaria was prevalent.
In the 1600’s, slave traders brought people from the African Gold Coast to the Americas. The slave traders sold some African people to plantation owners in the swampy malaria-infested Surinam. Africans were also sold to plantation owners on the malaria-free island of Curacao off the coast of Venezuela.
The effects of 400 years of natural selection upon people living in these different environments are now evident. Today the frequency of sickle-cell trait in the gene pools of the people of malaria infested Surinam and West Africa are relatively high. The trait has decreased in the African American gene pools of Curacao and the United States, which are malaria-free.
Question 4.2.
What do you predict would happen to the sickle-cell trait in the African gene pool and the American gene pool if malaria were completely eradicated?
IX. Protecting the Genetic Code
Our understanding of DNA has enabled us to unlock the molecular language of heredity. With the exception of identical twins, each individual’s DNA is unique. The aftermath of World War II was a grim warning about the vulnerability of our genes. The general public began learning about chromosomes and gene theory through the news coverage of birth defects and cancer in the children of Japanese people who survived the atomic bombs at Hiroshima and Nagasaki. With the advent of nuclear technology, humans now run the risk of contaminating large areas of the planet with radioactive materials that can severely damage genetic codes for thousands of years to come.
Concern for the vulnerability of chromosomes has stimulated extensive medical research related to cancer, genetic disorders, and environmental contaminants.
The Food and Drug Administration and the Environmental Protection Agency were established in response to growing public concern about hazards such as radiation, pollution, and chemicals in our diet. The 1978 evacuation of the residents of Love Canal, who were experiencing cancer and birth defects, alerted Americans to the dangers of unregulated chemical dumping.
The Environmental Protection Agency has identified thousands of toxic waste sites across the country which qualify for government clean-up under legislation known as the “Superfund.” You and your neighbors can now find out if there are any old toxic dumps in your area.
A superfund site
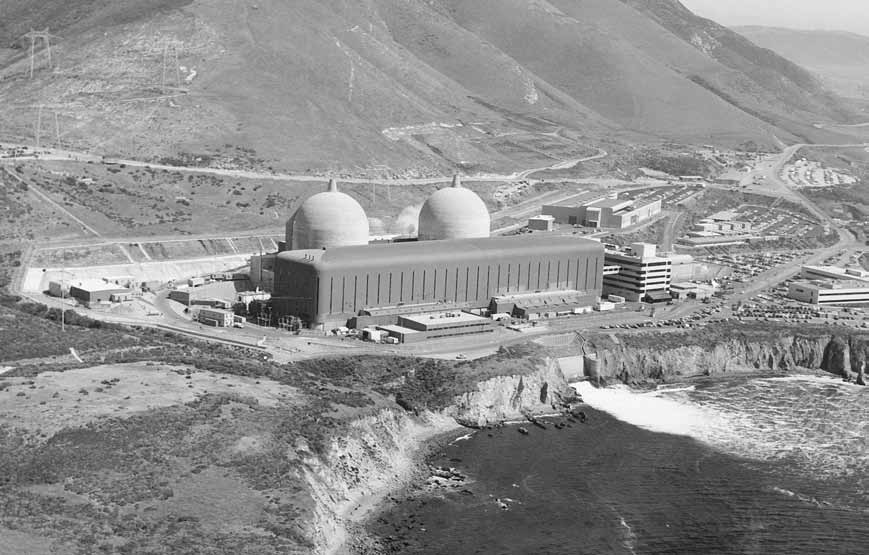
X. Genetic Engineering
With recent advances in genetic engineering, scientists can identify the location of genetic disorders on the chromosomes as well as the changes in the nucleotide codes causing the mutations. With the recent success in mapping of the human genome, it may be possible to repair genetic damage in humans as well as in endangered species using genetic banks that store samples from healthy organisms. The Human Genome Project web site provides a wealth of information about molecular research:
https://doe-humangenomeproject.ornl.gov/
DNA science is also providing tools for protecting biodiversity. It is possible to analyze tissue from an organism and produce a “DNA fingerprint.” Game wardens and law enforcement people are using DNA evidence to identify and prosecute poachers who illegally kill animals and sell them on the black market. In Japan, DNA “fingerprinting” is being used to identify protected species of fish that are illegally sold in restaurants and sushi bars.
Genetic engineering, like many advanced technologies, is highly controversial since it may be used in ways that may be either highly detrimental or highly beneficial. Some of the possible downsides to genetic engineering are:
- creation of species that would, if not contained in closed environment, compete with natural species,
- the effect of genetically modified crops on the environment and those crops’ role in agriculture systems,
- questions about rights of companies to patent newly created species, or specific genes, even human genes,
- risk of harm from genetically modified food and whether genetically modified food should be labeled,
- the role of government in regulating.
Potential benefits are also significant:
- gene therapy in medical treatment of a disease by repairing or replacing defective genes or introducing therapeutic genes to fight the disease
- creation new and better drugs such as bio-engineered insulin, hormones, and blood clotting factors.
- in agriculture, increase in the efficiency of photosynthesis, increasing the resistance of the plant to salinity, drought and viruses and also reducing the need for a nitrogen fertilizer, herbicide, or pesticide.
- bio remediation—cleaning up waste and pollution with the help of living organisms.
- bacteria that can transform waste into ethanol as a non-fossil fuel.
Genetic engineering also has the potential of creating new types of human beings with advantageous traits—an especially controversial notion.
XI. The Importance of Biodiversity in Food Crops
The continuing loss of genetic variation in food crops is a problem that may have severe consequences for human populations. Edible plants growing in the wild have survived a multitude of plant eaters and are rich in genetic variation. Modern crops however are genetically uniform and highly susceptible to rapidly evolving pests and blights that can quickly spread throughout agricultural regions causing massive crop failure.
The total number of important food crops is only about 130 species, most of which have been planted by people since the Stone Age. There appear to be twelve major areas around the world where a diverse gene pool for certain crops has been maintained in wild populations. Most of these areas of crop diversity are threatened by development such as the spread of cities, logging, and livestock grazing. For example, in 1977, scientists searching in Ecuador found a wild relative of avocado that was resistant to a disease called “blight.” This wild strain of avocado was limited to twelve trees in a tiny patch of forest that had somehow escaped logging. Another region of crop diversity closer to home is in our upper Midwest, where the blueberry, cranberry, Jerusalem artichoke, pecan, and sunflower originated. The value of genetic diversity has long been recognized by plant breeders, many of whom have helped establish seed banks to preserve the genes of wild crops.

Dedication to Diversity
“In the winter of 1942, the city of Leningrad was under siege, surrounded by Nazi tanks. For 900 horrifying days, the citizens endured artillery fire and aerial bombardment, but the worst was starvation. Before the blockade lifted, it had caused more than 600,000 men, women, and children to starve to death….At the Leningrad’s Vavilov Institute, a botanical and agricultural research center, thirty-one scientists remained to guard the unique collection of plants and seeds gathered meticulously from their places of genetic origin all over the world….Dr. Dmytry S. Ivanov, the institute’s rice specialist was surrounded by bags of rice when he was found dead at his desk. He was reported to have said shortly before his death, “When all the world is in flames of war, we will keep this collection for the future of all people.”
—From Earth in the Balance
by Vice President Al Gore, pg. 281, 1992.
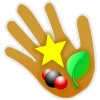
LB4.2. Investigation:
Deep Dive Into Genetics
Choose from resources below or find your own to explore more about the latest developments in genetics. Compose a synopsis of your findings to share with others.
- CRISPR: a powerful gene editing technology
- How CRISPR works
- CRISPR 101
- Innovative Genomics Institute – https://innovativegenomics.org
- Jennifer Doudna – https://vcresearch.berkeley.edu/faculty/jennifer-doudna
- University of California Division of Agriculture and Natural Resources Statewide Biotechnology Workgroup – http://ucbiotech.org – focused broadly on agriculture, crops, animals, foods and the technologies used to improve them.
- DNA Learning Center at Cold Spring Harbor – Students Talk Science
- Genetics Education Center, University of Kansas Medical Center – http://www.kumc.edu/gec/
- PhET online interactive about natural selection (rabbits and wolves)
https://phet.colorado.edu/en/simulations/natural-selection - Science Explained, The Cloning of Dolly – http://www.synapses.co.uk/science/clone.html
The discovery of how natural selection interacts with inheritance has shown us the importance of conserving Earth’s library of genes. The diversity of traits in a population makes it possible for a species to adapt to a change in the environment. The millions of species that are alive today contain an incredible variety of successful traits that have withstood the test of ice ages, meteor impacts, shifting continents, disease-bearing viruses and bacteria, and competing life forms. If a species becomes extinct, it is gone, and so is its unique combination of genes. The loss of its genes is also a loss of many potential new species adapted to a different Earth of the future.
In the next chapter we will investigate diversity in living systems that depend on soil resources. Because biological evolution is a relationship between genetics and environment, maintaining a variety of habitats is essential to conserving both species and genetic diversity within species.