CC2. What’s So Special About Carbon Dioxide?
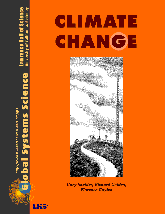
Chapter 2
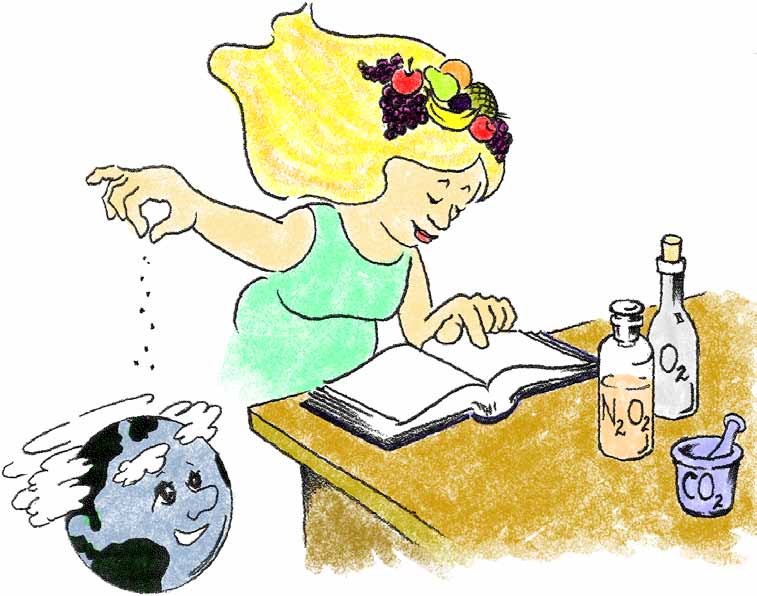
How does carbon dioxide trap heat? In short, the atmosphere allows the Sun’s visible light energy to pass through it unhindered and heat the ground. When the warmed land masses and seas cool, they radiate their energy back toward space in the form of infrared rays. Carbon dioxide in the air absorbs some of that infrared energy and sends a portion of it back to Earth, thus raising the temperature.
But why does carbon dioxide absorb infrared energy, while the far more abundant oxygen and nitrogen gases in the atmosphere do not? To fully explain how carbon dioxide and some other gases act to allow sunlight in and trap infrared energy we need to first find out how matter and energy interact.
I. Matter
For thousands of years people have been observing nature and trying to reduce its complexities to simple, understandable terms. Many cultures recognized that everything was made up of just a few categories of matter. The ancient Greek, Aristotle, who lived 2,300 years ago, wrote that everything is made of different combinations of four basic ingredients: earth, air, water, and fire. For example, he thought wood was made of earth and fire. The process of burning let the “fire” out of the wood, leaving the black solid “earth” behind.
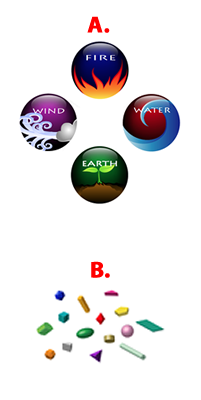
A different point of view was suggested by Democritus, a Greek philosopher who lived nearly a 100 years before Aristotle. He thought all things were made up of little indivisible particles (“atomos” in Greek), with nothing in between the particles.
A. Aristotle’s idea.
B. Democritus’ atoms
Democritus’ daring idea is very similar to the view virtually all scientists hold today, called the particulate theory of matter. According to this theory, all naturally occurring matter is made of only 92 different kinds of atoms. Materials made of only one kind of atom are called elements. An atom is the smallest unit of an element that still has the properties of that element. All atoms have the same general structure: a central nucleus, which has a positive electric charge, surrounded by negatively charged electrons.
Long ago, it was thought atoms were like little solar systems, with atoms whirling around the nucleus like planets around the Sun. As it is impossible to precisely locate any particle within an atom, today the image of a cloud is often used to show the unpredictable positions of the electrons.
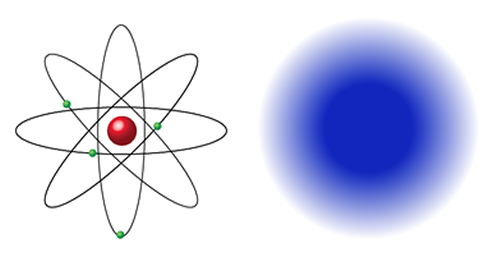
Materials made of combinations of two or more elements are called compounds. The smallest unit of a compound is called a molecule. Carbon dioxide is a compound made of one carbon atom and two oxygen atoms; so its chemical symbol is CO2.
Scientists frequently draw diagrams of molecules showing how the different atoms inside them are arranged. However, none of these representations are intended to show what an atom or molecule “really” looks like, but rather, to represent various properties we know about them.
Understanding what is so special about CO2 involves knowing about the interaction between matter and energy, so we’ll next discuss energy.
II. Light Energy
Energy comes in many different forms, but for the purposes of understanding what’s special about CO2, it’s most important to understand light and heat energy. First, we’ll consider light.
In the year 1666, at the age of 23, Isaac Newton began one of the first systematic investigations of light. He sent sunlight through a triangular piece of glass, called a prism, and found the effect to be “a very pleasing divertissement, to view the vivid and intense colors produced thereby.”
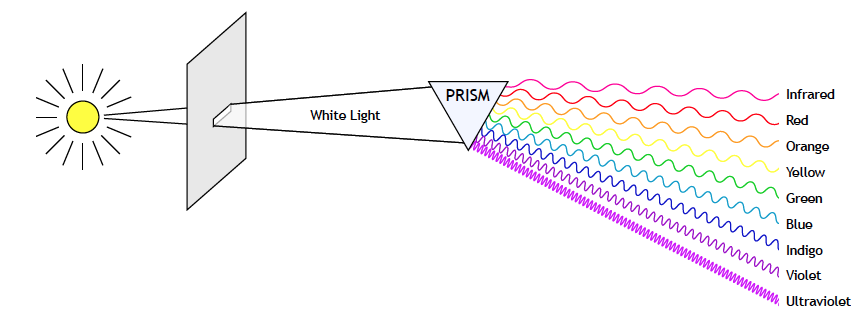
The glass prism cast elongated patches of light that were separated into bands of color: violet at one end, red at the other. In between was a continuous gradation of different colors. He noted the similarity of the pattern of colors to that of the rainbow. Newton named the pattern a spectrum.
Newton wondered where the colors came from. Did they come from the light or from the glass prism? He answered this question by inserting a second prism upside down into the beam. He found that if the prisms were close together, the colors combined again to form a bright beam of sunlight. Thus he concluded that the colors of the spectrum did not originate in the glass. His experiments showed that sunlight was composed of various colors and that the colors could be separated or combined with the help of a glass prism.
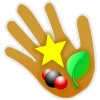
CC2.1. Investigation: Two Prisms
Try splitting a beam of light into its different colors, then combining the light again into a single beam—just as Isaac Newton did more than 300 years ago.
Two theories soon developed to explain the nature of these different colors: the particle theory and the wave theory of light. Newton favored the idea that each ray was composed of streams of tiny particles. He thought that some particles were blue and produced blue light, while other particles were red and produced red light.
Other investigators performed experiments showing that light acted more like a wave. Their idea developed into the notion that blue light was composed of waves that were shorter, from crest to crest, than red light waves. The distance between wave crests was called the wavelength. Each of the colors of the visible spectrum had its own specific wavelength.
According to the wave model, light of a given wavelength has a corresponding frequency. Frequency can be thought of as speed of vibration, or how frequently a wave occurs in a certain period of time. Imagine making waves in a long spring, like a Slinky. Shaking your hand faster produces very short waves with high frequency. Shaking your hand slower produces longer waves at a lower frequency.
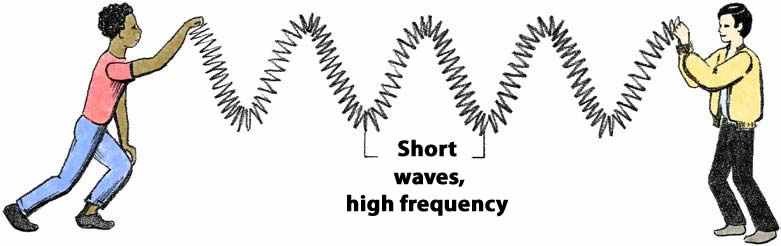
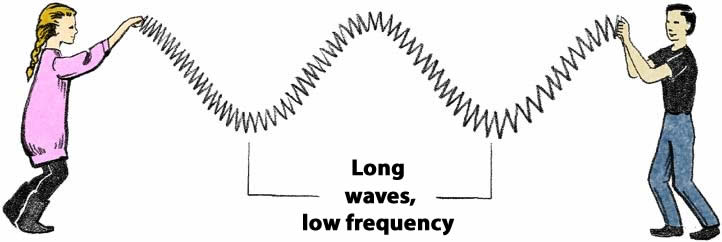
III. Infrared (Heat) Energy
In order to understand the greenhouse effect it’s important to know about infrared energy. Infrared energy was discovered more than 100 years ago when the English scientist Sir William Herschel used a prism to spread sunlight into a spectrum, and used a thermometer to measure the temperature in each color. He was amazed to find it was warm beyond the red end of the spectrum. He called the invisible form of energy, which could only be detected with the use of a thermometer, infrared rays.
If you have ever stood in front of a fire and felt its warmth, you have detected billions of infrared photons bombarding your skin. If someone or something gets between you and the source of infrared radiation, you can feel the radiation being blocked. Hold your hand near a rock or any other object that has been in bright sunlight for a while. You will feel the infrared waves as heat.
Objects that are warm radiate more infrared energy than objects that are cold. According to the molecular theory, this is because hot objects are composed of rapidly vibrating molecules. As the object cools, the molecules slow down. The energy from the vibrating molecules is converted to photons of infrared energy.
Warm objects that do not give off visible light can be “seen” with special instruments that detect infrared photons. Human beings and other animals, for example, radiate considerable infrared energy. The military makes use of infrared detectors in their night vision cameras and scopes. Some special photographic films are sensitive to infrared radiation and can take pictures of warm objects in the dark. Some snakes have sensors to “see” infrared light. This ability helps them locate prey at night.
Our skin nerve cells are not sensitive to visible light photons, but our eyes are supremely sensitive to them! The retina at the back of a human eyeball can distinguish photons of slightly different wavelengths and interpret them as different colors. As we lie on a beach, our eyes see the Sun’s visible light energy, while our bodies feel its infrared energy.
It is now known that infrared energy is the same kind of energy as visible light, but the wavelength is longer. The wavelength (or color) of an object depends on its temperature. You can see this effect with a light bulb attached to a dimmer switch. A clear light bulb will allow you to see the thin metal filament inside the bulb. When the electricity begins to flow the filament will glow a dull red. As the dimmer is turned up the filament will get warmer and warmer. It will gradually turn from red to orange to yellow, and eventually it will become white hot.
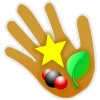
CC2.2. Investigation:
Infrared Energy and Your T.V.
Wireless remote control devices for television sets and video cassette recorders use invisible beams of infrared radiation (IR) as the means of transferring the information from the device in the hand of the viewer to the controls within the sets.
Try these experiments.
A. Measure the “cone” or area of infrared energy by putting the remote control device on a sheet of paper taped to a table. Move the table slowly away (and then closer) from the television. Mark the position where the device begins to work.
B. Even though you can’t see it, the beam of infrared light will reflect off a wall or the ceiling just as a beam of visible light will reflect off a mirror. Try controlling your TV set by “bouncing” the beam around the room, instead of pointing it directly at the TV.
IV. Carbon Dioxide—The Gatekeeper
Ideas about matter and energy come together as we return to the question, “What’s so special about carbon dioxide?”
Carbon dioxide acts as a sort of gatekeeper. As we said at the beginning of this chapter, carbon dioxide allows visible light to pass right by but will absorb infrared energy. The key to understanding how it does this is a concept called resonance.
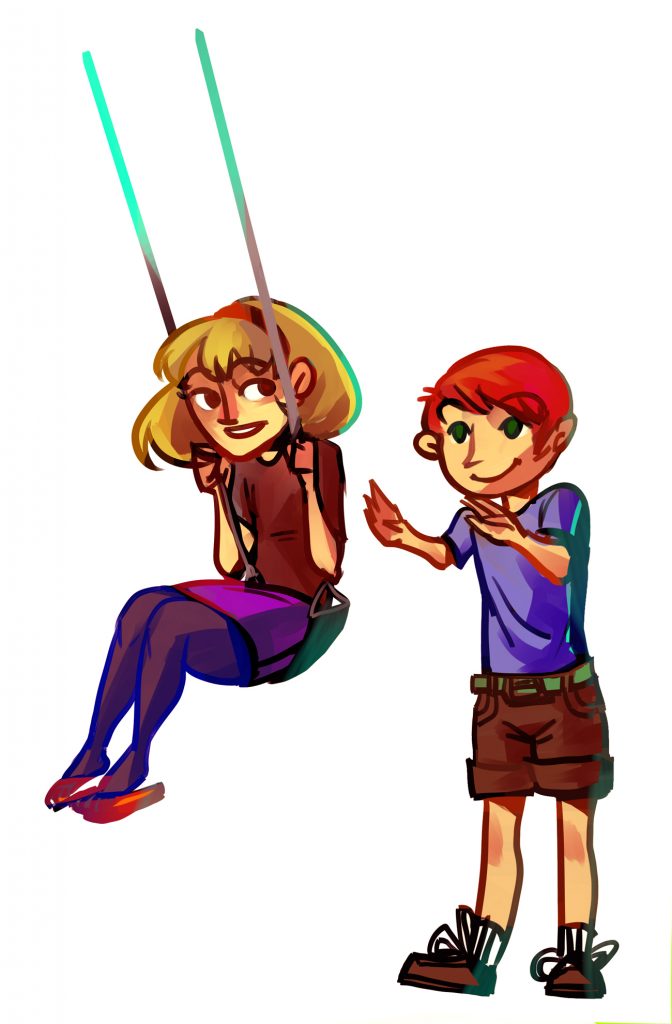
Imagine you are pushing your friend on a swing. If you want to get your friend to swing as high as possible, you need to time your pushes just right. If the swing goes back and forth once every second, you must push at a frequency of once per second. You must also give the push at just the right instant, when the swing is just about to go forward.
The right pushing speed required to transfer energy from one system (you) to another (the swinger) is called the resonant frequency. The following activity illustrates how resonant frequency is important when light energy interacts with molecules in the atmosphere.
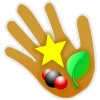
CC2.3. Investigation: Why Do Some Molecules Absorb Infrared Energy?
In this investigation you will experiment with models of molecules that are millions of times larger than the real ones to see what happens when they are energized with different frequencies of vibration.
V. The Resonant Frequencies of Real Molecules
In the previous experiment, you probably found some of your models responded to shaking at certain frequencies. If you vibrated your hand at the resonant frequency, the bonds between the atoms flexed in their natural rhythm, and the model absorbed your energy and “danced.” That is a good analogy of how molecules in the atmosphere respond when they are struck by sunlight. In fact, everything that is free to move—from baseball bats and bridges to electric circuits—has its own resonant frequency. If energy that matches the resonant frequency is put into it, the object will absorb that energy and start to vibrate.
The resonant frequency of an object depends on its structure. When you tune a guitar by changing the tightness of a string, you are changing the structure so the string will vibrate at a different resonant frequency. Each model in your experiment probably has a different resonant frequency because each structure is different.
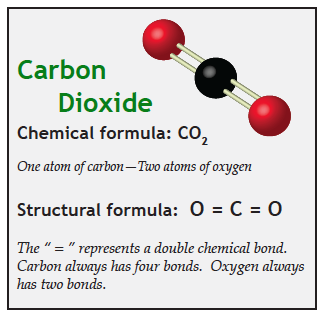
Now let’s transfer this idea of resonance to real molecules. Molecules are far smaller than objects like bells and guitar strings; so the resonant frequencies of molecules are much, much faster. They resonate at speeds comparable to the frequency of light, which is over ten thousand trillion times a second!
Photons of visible light vibrate too fast to affect any of the molecules in the atmosphere. That is the reason visible light goes through air. However, photons of infrared energy vibrate at just the right frequency to transfer their energy to molecules of carbon dioxide and methane, which causes those molecules to vibrate. We experience this vibration as heat.
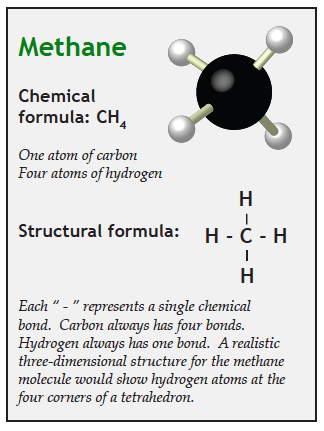
Gases that vibrate (and therefore heat up) when infrared energy passes through them are greenhouse gases. Oxygen and nitrogen are not greenhouse gases because they will not resonate at the frequencies of infrared energy. Carbon dioxide, methane, and water vapor are greenhouse gasses because they vibrate when they encounter infrared photons.
VI. Carbon Dioxide and Earth’s Greenhouse Effect
As you know, molecules of carbon dioxide, methane, and other greenhouse gases vibrate when struck by infrared photons, while oxygen and nitrogen molecules do not. It is that property that allows greenhouse gases to keep our planet warm enough to sustain life.
It is important to remember Earth’s greenhouse effect is not new. A certain amount of carbon dioxide, methane, and water vapor has been in the atmosphere for billions of years. If those gases were to suddenly disappear, our entire planet would enter the deepest, coldest ice age it has ever known, and most life would perish in a short time.
Global systems scientists are not worried about the natural greenhouse effect, but about an increased greenhouse effect due to human activities. It is therefore very important to find out how quickly the concentrations of greenhouse gases are increasing in the atmosphere.
Although water vapor produces most of the heating in our atmosphere, carbon dioxide is the most important of these gases because it is an unavoidable side effect of industrial society. Cars, trucks, and buses produce carbon dioxide whenever they burn gasoline. Most of the world’s electrical power plants add huge amounts of carbon dioxide to the atmosphere every day as they consume trainloads of coal or oil. As developing countries modernize by building power plants and factories and more and more people have cars, more and more carbon dioxide is added to the atmosphere, increasing the greenhouse effect.
In 1957 a laboratory was set up to continuously monitor the amount of carbon dioxide in the atmosphere. Because carbon dioxide levels are very high in cities where there are many automobiles and factories, the laboratory had to be located as far from these sources as possible. An ideal location would be high in the atmosphere, far away from large cities and industrial regions.
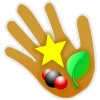
CC2.4. Investigation:
Getting A Piano To Sing To You
If you have access to a piano, try this experiment. Have a friend hold the “sustain pedal” down so that the strings are free to vibrate. Position your head above the strings and sing a musical note into the piano toward the sounding board beneath the strings. The sounding board will reflect the vibrations. Some of the strings will have natural resonant frequencies that match your input. Only those strings will vibrate. Listen for them. The piano will sing your note back to you.
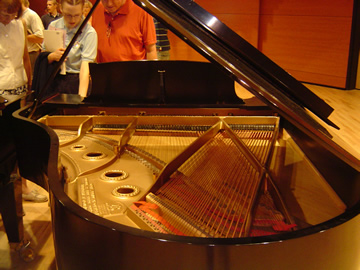
“Piano,” © 2005 Halley, used under a Creative Commons Attribution-ShareAlike license: http://creativecommons.org/licenses/by/2.0/deed.en