EC1. Earth Alive!
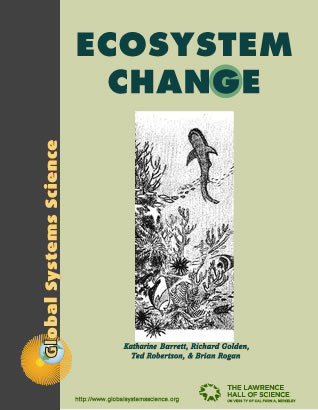
Chapter 1
Imagine you are an astronaut viewing planet earth from a space shuttle 160 miles above the surface.
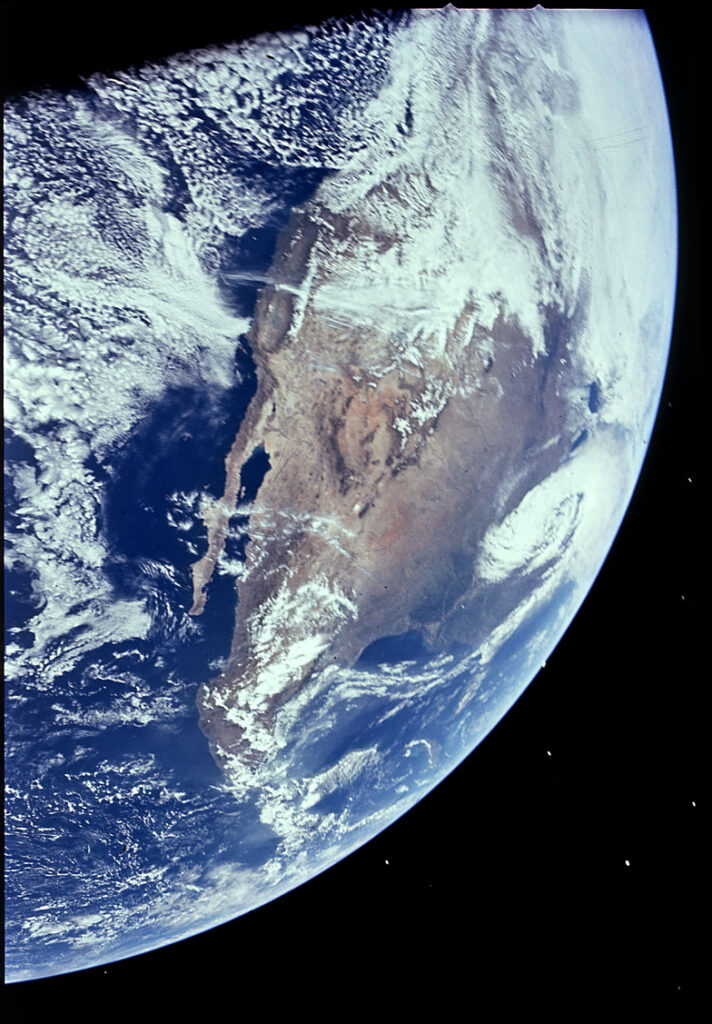
Changing patterns of clouds and reflections from water and snow fields create the illusion of a colossal spherical organism. Many human cultures have believed that Earth is alive; many have attributed human qualities to the planet. The ancient Greek goddess of the Earth was called Ge or Gaia (Guy-ah). It was from this name that the words geography and geology were formed.
In 1978, J.E. Lovelock published his book Gaia: A New Look at Life on Earth. He begins by pointing out that, “Journeys into space did more than present the Earth in a new perspective. They also sent back information about its atmosphere and its surface which provided a new insight into the interactions between the living and the inorganic parts of the planet. From this has arisen the hypothesis, the model, in which the Earth’s living matter, air, oceans, and land surface form a complex system which has the capacity to keep our planet a fit place for life.”
This controversial idea, now called the Gaia Hypothesis, has sparked new research focusing on the interactions between Earth systems. The process of supporting or refuting a new idea such as this has led to many important discoveries about life. As you read this book, make a list of evidence related to this scientific debate about whether or not Earth operates as a single organism with the capacity to regulate conditions for life. We encourage you to critically examine this idea as you learn more about the systems and interactions operating in nature.
I. Living Systems
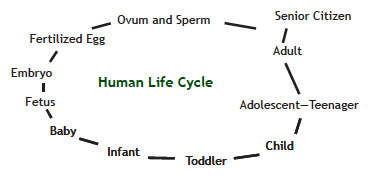
Organisms are living systems having life cycles that progress from spores, ova or eggs to mature adults that reproduce new offspring. These reproductive systems or cycles can be quite complex and involve other organisms as sources of food or shelter. If the system of reproduction breaks down at any point in the cycle, the organism fails to perpetuate its kind.
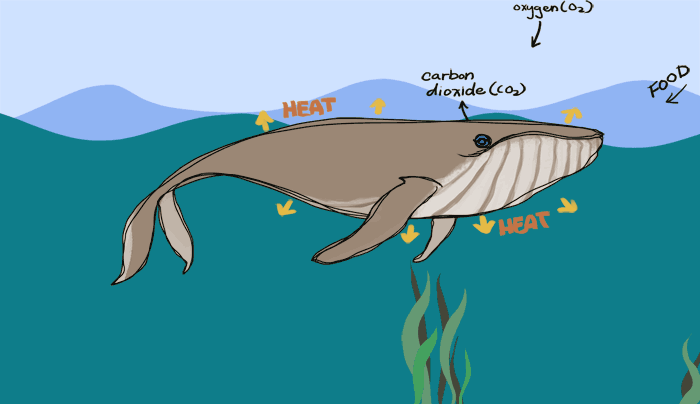
Living systems can be large like a whale or ocean, and microscopic like an Amoeba. Large systems usually include many subsystems that interact with each other in a particular way.
Microscopic systems such as a Paramecium and a blood cell have subsystems within them that interact, consuming and producing things.
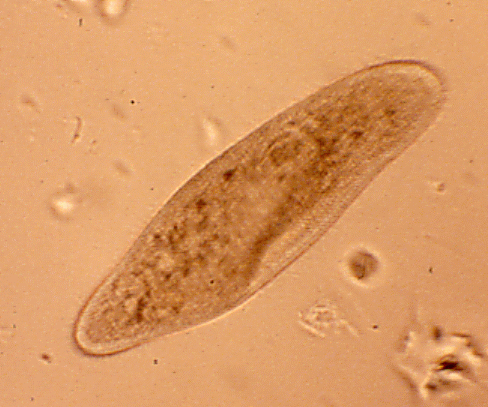
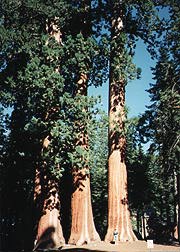
It is hard to imagine a gigantic sequoia having single-celled ancestors, and yet each subsystem in the tree depends on other systems to provide or consume substances and trap or release energy. For example, a leaf is made up of different kinds of cells that have different jobs. Some cells control the openings in the leaf, letting carbon dioxide gas enter and leave. Cells that form the veins strengthen the leaf and transport water and food to other parts of the tree. Green pigmented cells capture light energy and transform it into food.
Sequoia
Biologists have been working for centuries to identify and understand the amazing variety of life forms on Earth. The science of ecology has grown out of attempts to understand the relationships between organisms and their surroundings. Eco comes from the Greek word oikos or house, and ology, is Latin for to study. This area of science brings together research from Earth science, physical science, and life science to piece together information about our planet.
An ecosystem, or “house system,” is a community of living organisms interacting with its environment in an area that has similar conditions such as climate and physical features. It can cover a large area like a rain forest, or be small like a pond in a city park. An ecosystem includes all of the organisms in an area and their non-living components such as rock, soil, dead organisms, water, air, and sunlight. All of the Earth’s ecosystems together make up larger areas called biomes, that share common plant, animal and climate characteristics, such as a desert or a tundra, and they group into the largest system we call the biosphere (life sphere), or the entire area which supports life on Earth.
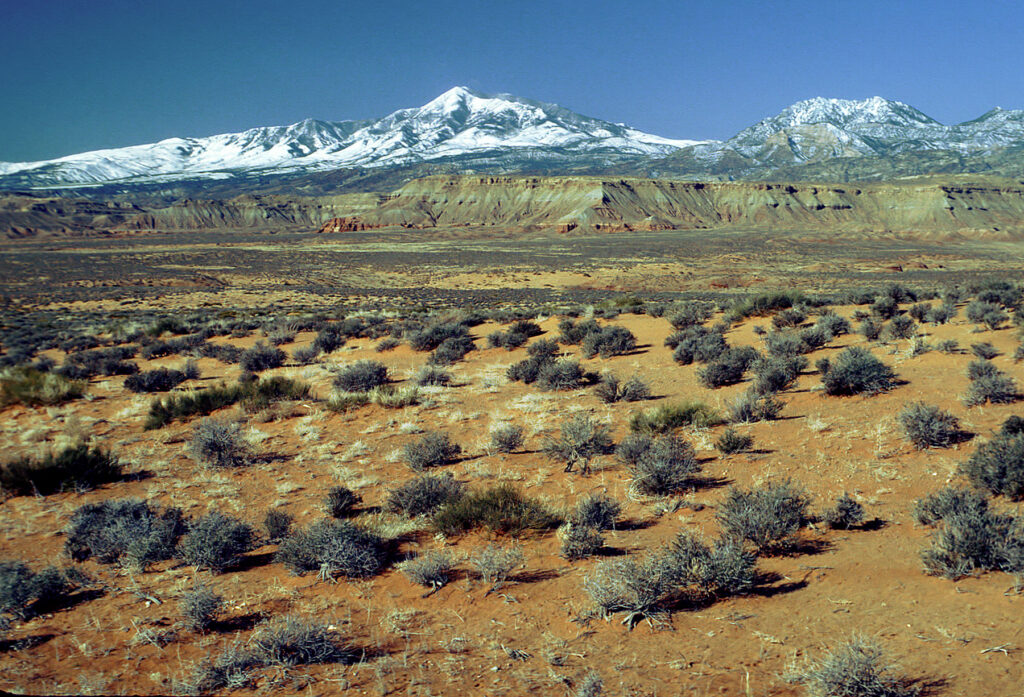
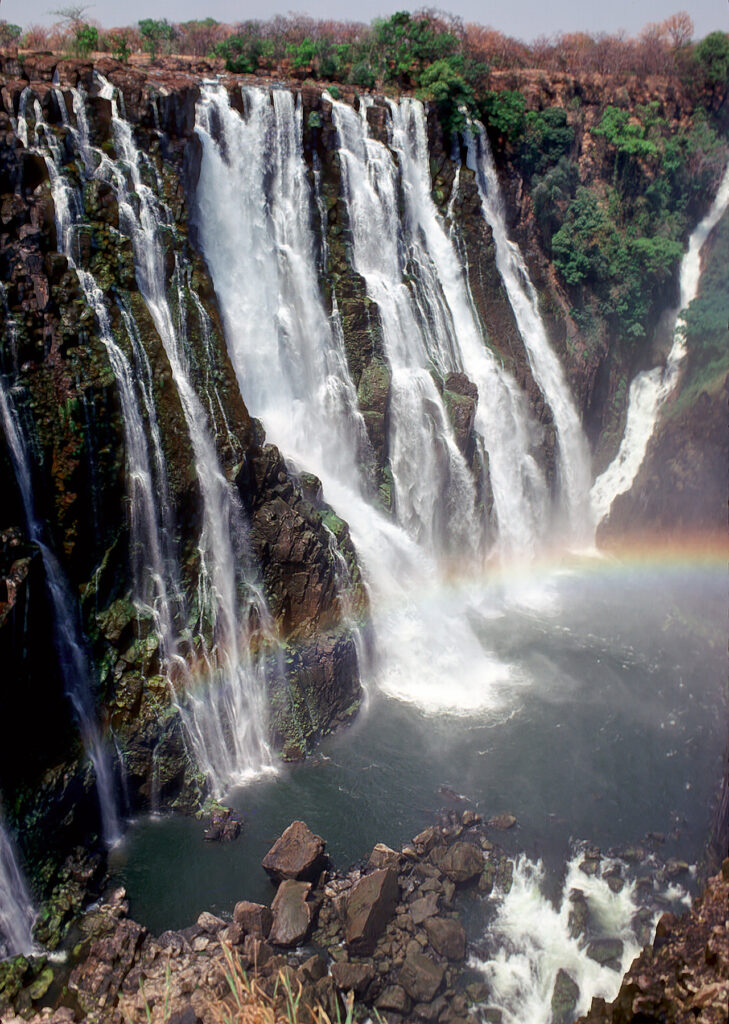
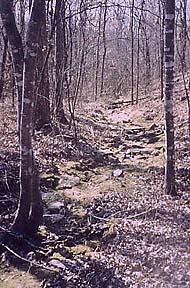
Deciduous forest biome
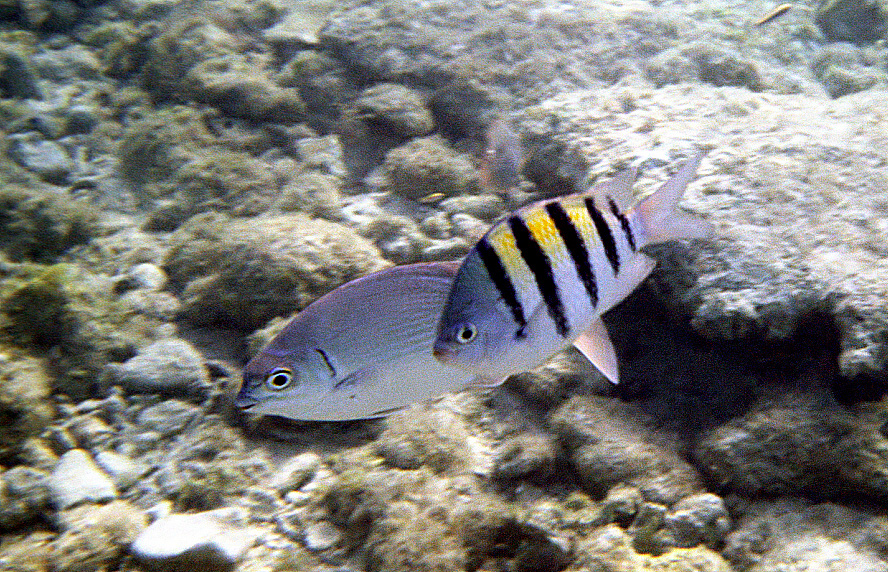
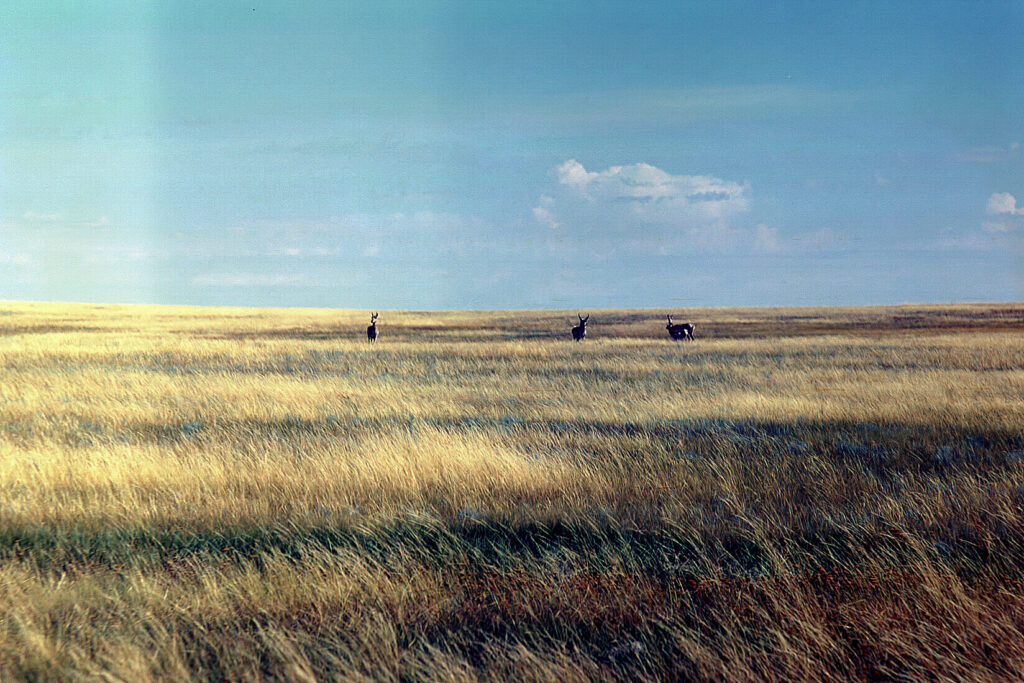
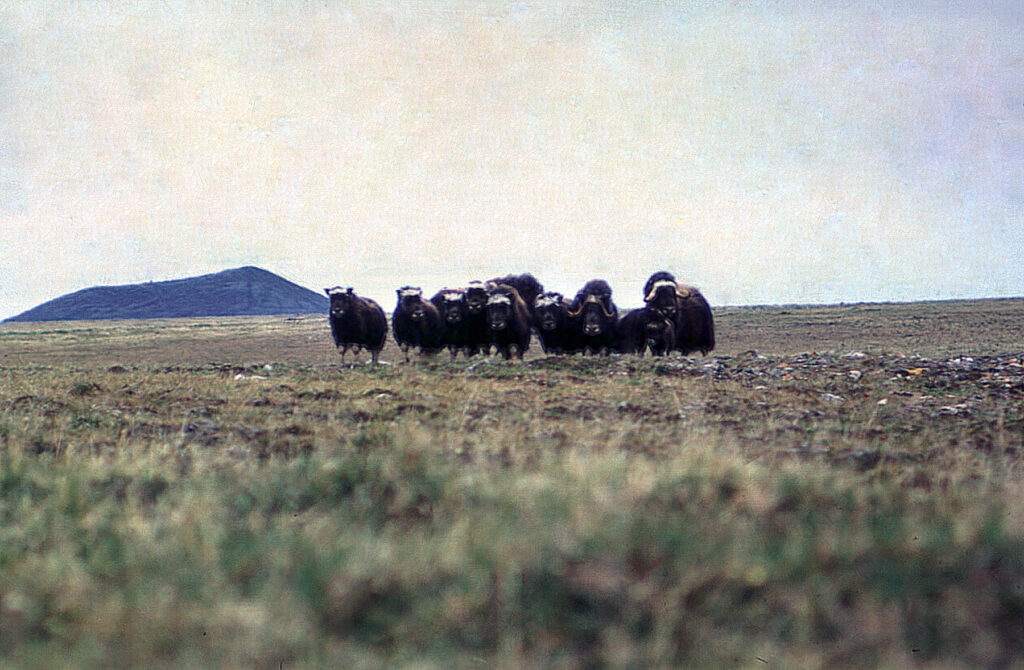
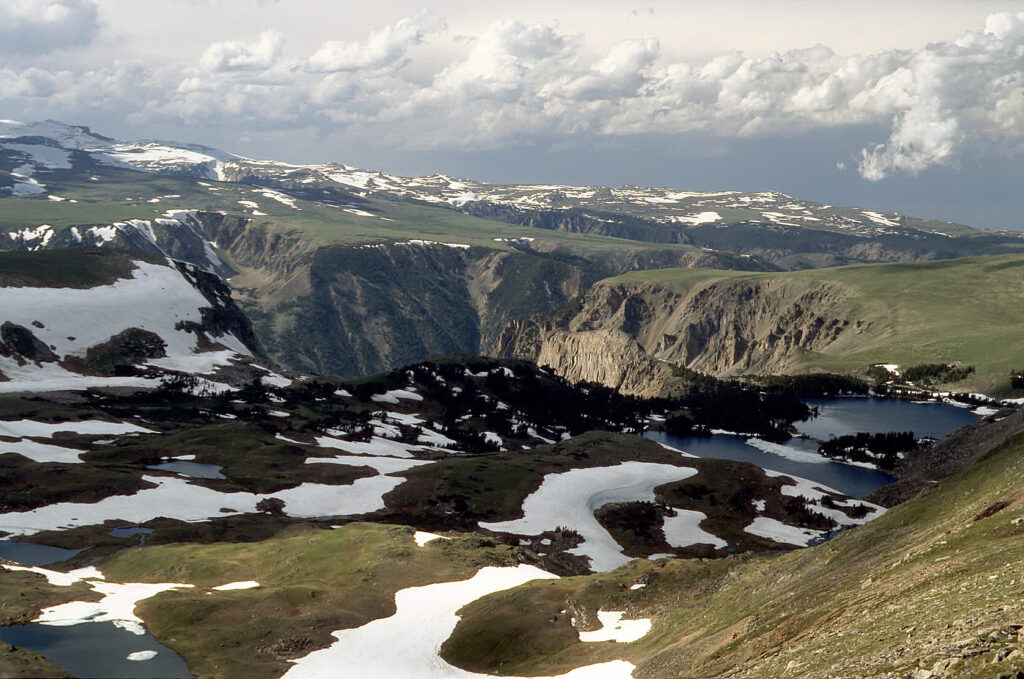

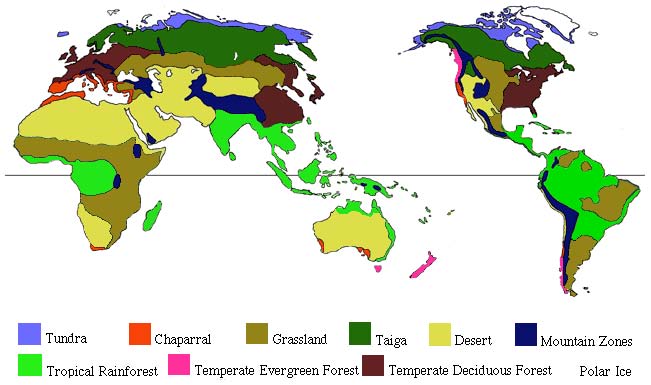
II. Everything’s Connected to Everything Else
When we start to look at the world as a vast array of interlocking systems we begin to understand the saying of ecologists, “Everything is connected to everything else.” There are systems all around us. Just look for the parts of the system and how they are connected, and you’ll begin to see how the system works.
Unfortunately, analyzing natural systems is easier said than done. In this chapter we’ll introduce two tools that you will use throughout the Global Systems Science course to understand our planet: systems diagrams and the concept of feedback. To show how these tools are used, we’ll introduce them in the context of an especially interesting part of our planet that is currently undergoing rapid change—the tropical rain forests of the world.
III. Example of A System: The Tropical Rainforest
Every forest is complex and tropical rain forests are marvelously so. Imagine exploring a patch of forest about the size of a couple of football fields with a guide to trees. If the forest is in North American you will be able to identify 10 to 15 different species of trees. But if you were to explore a patch of the same size in an Amazon rain forest, you would be able to identify as many 230 different species of trees, along with a very large variety of different plants and animals.
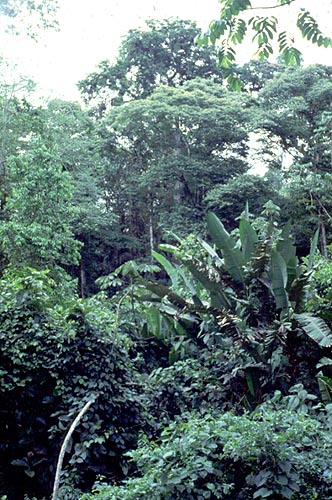
Rainforest in the lowlands of New Guinea.
Photo courtesy of ACT/Indiana University
Creating a diagram of such a system in detail is an impossible task because many of the interactions are still unknown. But it is sometimes useful to draw a simple map that shows just a few of the most important connections that we do understand.
For example, below is a diagram showing that water is recycled by plants. Notice that it is composed of boxes and arrows. Boxes are usually used to represent the total amount of something. Arrows represent changes or movements of the things in the boxes. In this diagram, the arrows represent the flow of water, and the boxes represent places in the system where water is found.
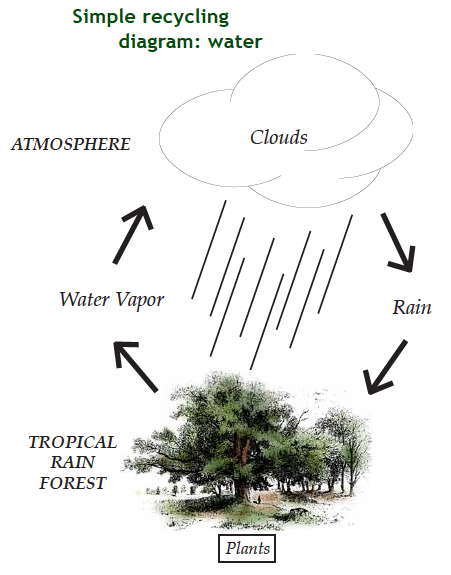
As in many systems diagrams, the water recycling diagram shows a loop, or cycle, which indicates how a certain material is recycled over and over again. In this case, the diagram shows a simple version of the water cycle. Other diagrams can be drawn to show how oxygen, carbon, nitrogen, or other materials are cycled through a healthy rain forest ecosystem.
For millions of years nature has maintained the rain forests by recycling water, carbon, oxygen, and other materials. When human activities interrupt natural feedback cycles the results can be devastating for the rain forest ecosystem. Today, for example, in many parts of the world, rain forests are burned so that the land can be used for farming.
When a rain forest is burned, water is no longer recycled by the active processes of trees and plants. That means the water vapor from the once-forested area is reduced. Fewer clouds form, and there is less rain.
That is not the only change that occurs. The rain that does fall washes the existing nutrients out of the soil. The rich topsoil is carried in muddy rivers to the sea.
For these reasons, farmland that was created by the burning of rain forests fails to produce good crops after a few years. The once-rich farmlands are then used to pasture cattle for a few more years, and when the fertility of the land is reduced even further, the land is abandoned and new forested areas are burned.
In the past, when native peoples burned the land to plant crops, the forest would eventually “reclaim” the land, and most of the plant and animal species would repopulate abandoned fields. But with the vast growth of the human population in recent decades, rain forests that have been replaced by farms and pastures soon become roads and settlements, so the size of the rain forest becomes smaller and smaller each year.
The World Resources Institute has estimated that an amount of rain forest equal in size to the area of the state of Washington is burned or chopped down every year. If the current rate of rain forest destruction continues, the rain forests of the world will be completely gone in less than 100 years.
IV. What Is Feedback?
On the previous page we used the term “cycle” to describe the ways that water and other materials are continuously recycled in a healthy rain forest. But the term “feedback loop” can also be used to describe what is going on. In the language of systems, feedback means that the output of a system automatically controls the input.
This is slightly different from the way we use the term feedback in everyday language—as when someone asks you, “How did you like the party? I’d like your feedback.” Suppose you answered, “There were too many people at the party!” From a systems viewpoint, the response would be considered feedback only if your friend really listened to you and decided to limit the number of people who were invited to the next party. Then the output (your experience at the last party) would control the input (the number of people who get invited to the next one.)
The main difference between the way we use the term “feedback” in ordinary language and in science is that in science the output controls the input automatically.
A room thermostat can be used to illustrate the principle. A wall-mounted thermostat senses and controls the heat in the room. The desired temperature can be set with a knob.
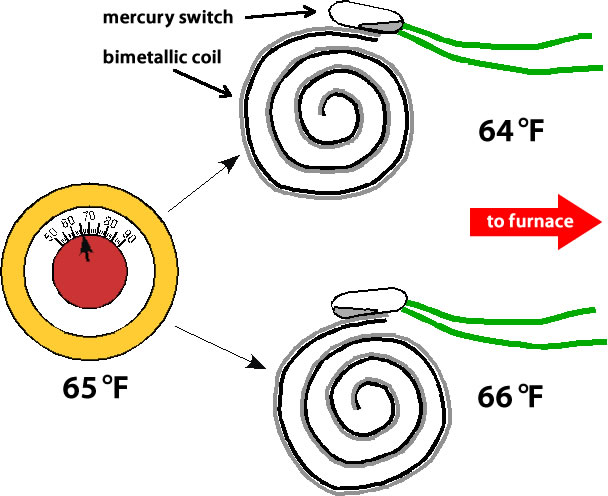
When the temperature of the room increases above that level, a coil of metal inside the thermostat bends and opens a switch. That automatically shuts down the furnace. When the temperature falls below a certain level the metal coil bends back and automatically turns the furnace back on, so the temperature of the room stays within a degree or two of the desired level. The temperature of the room is the output. It controls the heat flowing into the room.
In a tropical rain forest, rain is the input, and water vapor rising from the forest is the output. The feedback loop of falling rain and rising water vapor keeps the rainfall at about the same level year after year.
When a rain forest is burned, the feedback loop changes. Rain water that used to be absorbed by the plants and returned to the air runs off into rivers instead, sometimes causing floods downstream. That means less water vapor is returned to the air above the forest. Less water vapor means fewer clouds and reduced rainfall.
V. Negative Feedback
There are two kinds of feedback—negative and positive. Like the term feedback itself, these two kinds of feedback are sometimes misunderstood because the common meanings of the words “negative” and “positive” get in the way. We are accustomed to thinking of something negative as bad, and something positive as good. But there are positive and negative numbers that have nothing to do with good or bad. Positive and negative feedback also do not necessarily mean good or bad.
In negative feedback the change to the system is always reduced. The operation of the thermostat is a good example of negative feedback because any change in the temperature of the room is reduced by the action of the thermostat. If it gets too hot, the thermostat turns off the furnace and lowers the temperature of the room. If it gets too cold, the thermostat turns on the furnace and raises the temperature. Negative feedback tends to maintain systems at proper working levels.
Another example of negative feedback is temperature control within our own bodies. Imagine that you are running laps around a track.
The steps in a negative feedback loop for a runner
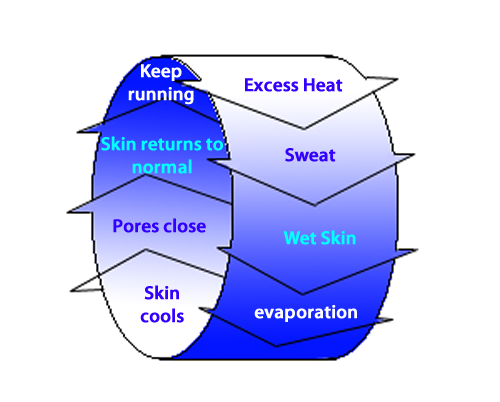
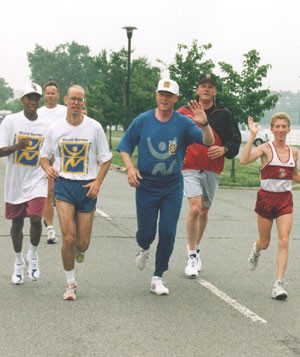
Your body heats up by the time you complete the first lap. If you are running fast, by the second lap your body temperature may rise dangerously. A control center in your brain sends a signal to the pores of the sweat glands in your skin to open and water is released. The evaporation of sweat carries heat away and your body begins to cool. If you continue running the sweat glands will continue to do their job of cooling you off. When you stop running you’ll feel a chill as the remaining sweat evaporates. The control center in your brain senses the cooler skin temperature and sends a signal for the pores to close. In this way your body temperature is maintained within a range that will not be dangerous. If you were out in the desert without water to drink this negative feedback system could break down. Without the cooling of water evaporation from your skin your body would overheat and you would collapse from heat exhaustion.
If you think about it, many of our body systems are controlled by negative feedback.
Question 1.1.
Can you explain how the amount of light coming into your eye controls how much more light comes in? Or how the amount of food you eat controls how much more food you eat?
VI. Positive Feedback
In positive feedback, the change to the system is increased. An example of positive feedback is an argument between two people. When a hateful remark evokes a response that is full of hate the response can be an even stronger hateful remark. The level of hate can grow until violence results. When this process occurs between nations, we call it “escalation of hostilities,” and war may result. Whereas negative feedback tends to maintain systems at current levels, positive feedback tends to cause systems to break down.
An example of a positive feedback in nature is the thunderstorm. When the energy from the summer sun warms the ocean, water molecules absorb that energy and evaporate, becoming water vapor. The air is also heated and it expands. A bubble of hot air containing large amounts of water vapor floats aloft. As the bubble of moist air rises, it expands and cools. The water vapor within the bubble condenses into droplets. It is then that we see the white fluffiness of a summer cloud.
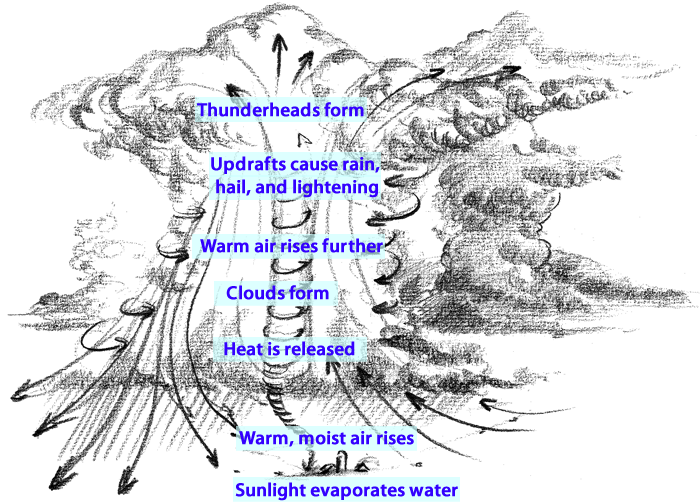
When the water droplets condense they release the heat they absorbed when they evaporated. This heat causes the air bubble to expand again and the bubble floats upward with increasing speed, becoming a huge cloud called a “thunderhead.”
The energy of the thunderhead system increases as more bubbles of air float upwards, condensing into clouds and releasing more heat. The intense updrafts may increase further if the storm drifts over land, where the surface is warmer.
Throughout the growing storm system, water molecules come together to form raindrops and begin to fall. Updrafts can swirl them up again and they can freeze into hail in the higher, colder air. Sometimes they increase in size by making many trips up and down, picking up more water vapor as they fall, and freezing again at higher altitudes. The energy of the moving air masses separates charged particles, causing lightning flashes to leap from cloud to cloud.
In a thunderstorm, the output of the system is the mass of rising air. The further it rises, and the more water condenses, the more heat is put into the system. This input makes the air rise further and faster, producing even more heat. This is positive feedback. And like all positive feedback processes, it can’t last forever. Eventually the rain and hail become too heavy to be sustained aloft and they fall with tremendous force. With its energy spent, the thunderstorm is over.
Although the thunderstorm system breaks down, the larger system of which it is a part continues to function. The hail melts, and the tremendous amount of water deposited by the storm flows into rivers and streams, watering forests and croplands as it returns to the sea.
In global systems science, the terms positive and negative feedback do not necessarily mean good or bad. They are two different kinds of natural processes that we need to understand if we are to determine how people are affecting those systems, and what it will mean for the future habitability of our planet.
VII. Using Models of Living Systems
Trying to study what happens in an ecosystem, never mind a biome which is very large, is difficult. Scientists use models to better understand the interactions between the parts of a system. Often, they cannot make a duplicate of what they are building. Using models, they avoid manipulating the environment in a way that might harm the organisms living there. For example, mechanical engineers test smaller, model oil rigs in wave tanks, and population biologists use computer models to predict trends in plant and animal populations.
In their quest to understand the relationships between natural systems such as a forest, scientists have turned to laboratory models. This new area of research known as synthetic ecology involves making artificial small-scale ecosystems. These systems, while small, duplicate many of the factors that effect life in a community.
Perhaps the most famous and controversial artificial ecosystem is the Biosphere 2 project in the Arizona desert near Tucson, is one of the largest living laboratories in the world.
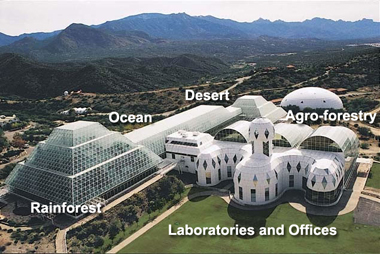
It is an airtight greenhouse covering 3.15 acres and 7.2 million cubic feet of volume. Within Biosphere 2 are several different biomes, which researchers use to experiment on Earth systems on a relatively large scale: a rainforest, a million-gallon salt water ocean, a coastal fog desert, and four other wilderness ecosystems.
In September 1991, four men and four women entered an eight-story greenhouse enclosure that covers an area the size of three football fields. Their goal was to remain and be supported in the enclosed system, independent from the outside world for two years. While the early results were mixed, they gave scientists many new insights into the complexities that underlie living systems. In 1994 Biosphere 2 was converted from an experiment to test the feasibility of humans living in a closed ecosystem to a large scale ecological laboratory and Western branch campus of Columbia University.
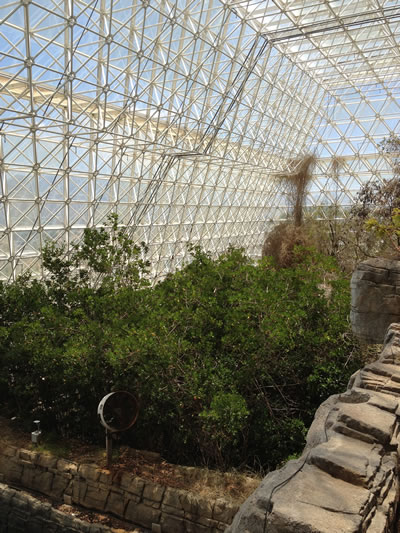
Photo by Alan Gould.
Biosphere 2 has the capacity for sensing and controlling the environment within. Sensors monitor the vital statistics of the environment including temperature, light, humidity, carbon dioxide and other qualities of the air and soil. In response to these readings, operators can control many of these variables by controlling rainfall, temperature and even carbon dioxide levels to mimic different environmental conditions.
Biosphere 2 is the only laboratory in the world where a mature grove of cottonwood trees can be grown under controlled conditions permitting researchers to examine the effects of different levels of carbon dioxide on the growth of the trees.
We invite you to explore the concept of systems while designing your own small ecosystem.
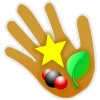
EC1.1. Investigation:
Make a Model Ecosystem
Design your own miniature ecosystem in a clear plastic or glass container and observe it for at least one month.
VIII. How Good Are Models?
Models are merely a substitute for conditions in the real world. Unfortunately, they can’t tell scientists exactly what will happen, but they can give important clues to some possible consequences if an important nutrient is removed or if a structure isn’t built with proper materials. Models give scientists a glimpse of what the future might hold. For us, they will be very important towards understanding what might happen if we change our environment.
The Biosphere II model was fraught with controversy. The original purpose of the model was to sustain the participants within the enclosure for a significant period of time where everything they needed would be provided by the system. However, problems quickly arose; atmospheric gas levels were artificially changed when oxygen began to run out and participants left and returned to the biosphere, contrary to the original goal of being a self-contained community. While this sparked outrage in the scientific community that it wasn’t a valid study, the project nevertheless taught scientists a lot about the difficulties in trying to duplicate the conditions that occur in environments on Earth.
IX. Conclusion
Trying to understand how ecosystems work is a difficult task. When scientists try and change one factor another is affected. As we have seen, every organism is dependent on every other organism. Models are one way to try and understand them but they are imperfect. In the following chapters, we will look at how energy moves through the system and what happens when the ecosystem is drastically changed.