OZ8. Measuring Ozone
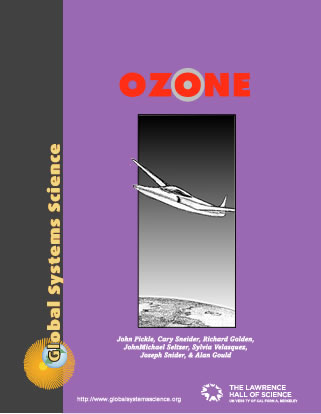
Chapter 8
{ Ozone Contents }
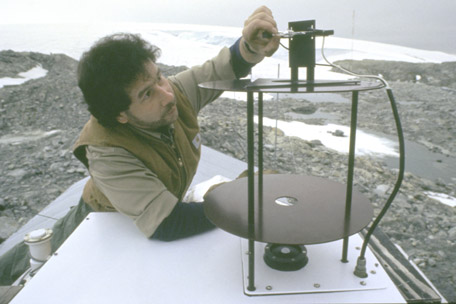
(Photo by Ann Parks Hawthorne, courtesy of the National Science Foundation.)
Accurate measurements of stratospheric ozone identified the ozone problem in the mid-1980s.
Accurate measurements are still needed to learn more about the complicated atmospheric chemistry involved and to monitor how the ozone layer responds as CFCs are phased out. The longest continuous record of ozone measurements extends almost 80 years. But despite this long data record, certain questions about the behavior of tropospheric and stratospheric ozone could not be answered from this one type of measurement. Because stratospheric ozone is so far above the Earth’s surface, advances in transportation and communication technology were needed to take the instruments to where the ozone was located.
I. Ground Stations
Since 1881, when Hartley and Cornu discovered the unexpected loss of UV section in the solar spectrum and related this to a possible ozone-rich layer somewhere in the atmosphere, scientists realized that by measuring the UV radiation, they could learn about ozone. However, it wasn’t until the invention of Dobson’s spectrophotometer in 1924 that repeatable, accurate, and precise UV measurements were possible. Measuring intensities of several wavelengths of UV from both direct and reflected sunlight, these spectrophotometers could provide both total column ozone amounts and vertical distributions of ozone concentration, which showed that the altitude of the maximum concentration of ozone was much lower than thought.
The station using a Dobson spectrophotometer in Arosa, Switzerland, has been collecting data since the mid-1920s, which is the longest continuous record of ozone measurements. Although new, more sophisticated techniques to measure ozone have been invented, these consistent, long-term (and safe) measurements from the same location are very valuable. Because of their established data records, Dobson spectrophotometer measurements are often used to calibrate data obtained by other methods, including satellites. Unfortunately, since the technique depends on natural light sources coming through the atmosphere, the measurements are strongly affected by aerosols (tiny, naturally occurring atmospheric particles, such as salt particles and dust) and pollutants in the atmosphere.
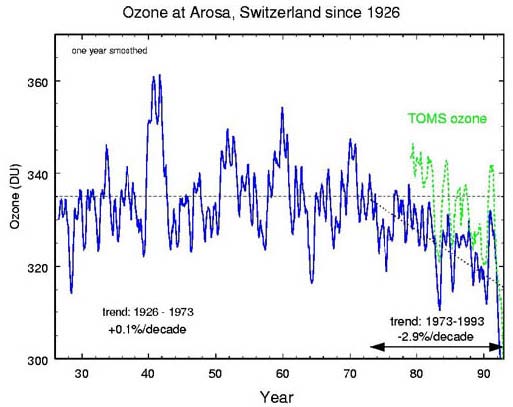
Long term data from Arosa, Switzerland, indicates that the consistent loss of ozone began in the 1970s.
(Graph courtesy of NASA.)
A second ground instrument was developed to measure chemical constituents in the atmosphere: the Light Detection and Ranging (LIDAR) instrument. Lidar is similar to radar (Radio Detection And Ranging) and can be thought of as a laser radar. Although radar was invented 1924, it wasn’t until after World War II when technology developed that made lidars possible. A lidar instrument projects two laser beams into the atmosphere—one with a wavelength of light that is absorbed by ozone and one that is not absorbed by ozone. The instrument measures the light that is reflected back towards the instrument. By comparing the two beams the instrument measures the concentration of ozone.
Lidars are able to measure continuously, compared to the Dobson spectrophotometer, which needs a source of natural light. The disadvantage of lidars is that they are very expensive and require significant expertise to maintain and operate. Current research is focused on creating automated, low power lidars that will be placed in remote locations around the globe.
II. Airbourne Measurements
Airborne vehicles, such as balloons, airplanes, and rockets provide the opportunity to carry instruments directly to the area of interest in order to make measurements. These measurements are the most accurate possible, since they can involve direct samples of ozone. However, there are limitations—the measurements are made only over localized regions and cannot provide a global picture of ozone distribution. There is also a high cost of human involvement.
Balloons
Balloons have been used for a long time to carry instruments or people into the sky. The first human flight took place in a hot air balloon in Paris in 1783. The radiosonde, a helium-filled balloon carrying weather instruments that transmit information to ground observers by radio, was first tested in the early 1930s, and the first U.S. radiosonde network was established in 1937. By 1960, ozone-measuring equipment could be packaged with the standard radiosonde equipment to create the ozonesonde. These instruments can measure ozone concentration up to altitudes as high as 40 km before the balloon bursts.
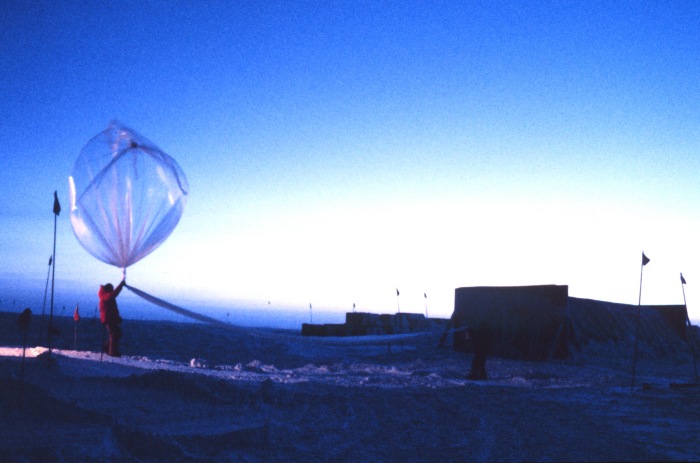
Launching an ozonesonde in Antarctica.
(Photo by Lt. Mark Boland, NOAA Corps, courtesy of NOAA Photo Library.)
Many lightweight devices have been invented to measure ozone from balloons. Most common are several varieties of devices that measure the electrical current produced during chemical reactions between atmospheric ozone and potassium iodide that is carried aloft. Another type of ozonesonde carries a laser aloft and measures the absorption of laser light projected from the balloon and reflected back to the sensor from a mirror hanging beneath it.
Ozonesondes provide detailed information on the vertical distribution of ozone throughout much of the stratosphere. However, since balloons are unpowered, the location of measurements cannot be controlled. Also, there are problems with the quality and comparability of the ozonesonde data resulting from the variety of sensors being used around the globe and the diverse calibration and operating procedures each country has adopted for the ozonesondes under their responsibility.
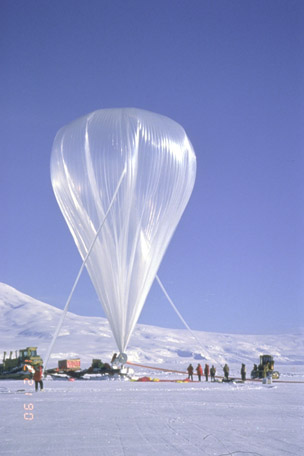
This 29-million-cubic-foot balloon being launched by the National Scientific Balloon Facility near McMurdo Station takes a 4,000-pound research payload to 38 km altitude, where it will circumnavigates Antarctica; staying aloft 8 to 10 days.
(Photo courtesy of the National Science Foundation.)
The term sounding comes from the maritime practice of calculating the depth of water with a weighted line, called a sounding line. The term is used today to describe many kinds of remote measurements that have a vertical component to the measurement, such as the vertical temperature profile (also called a temperature sounding). A sonde is an instrument that measures a sounding in the atmosphere or in the oceans.
Aircraft
Airplanes are used to make detailed measurements of ozone levels and related chemicals in the troposphere and lower stratosphere. Airplanes can carry a large number of instruments capable of measuring ozone, chemicals related to the production and destruction of ozone, and atmospheric conditions that affect ozone. Large airplanes, like NASA’s DC-8, can carry many people to perform sophisticated experiments.
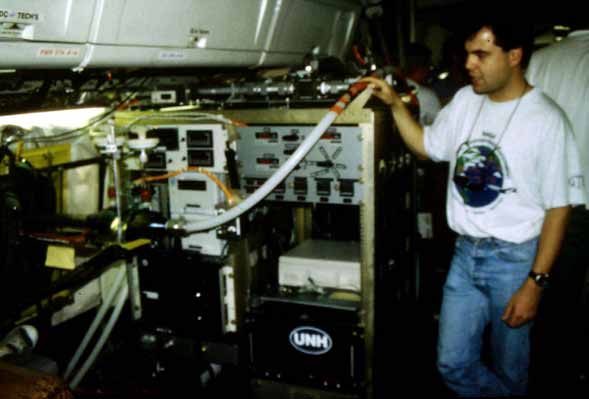
One instrument in place on the DC-8. The hose delivers air from outside to the instrument for measurements.
(Photo by Cary Sneider.)
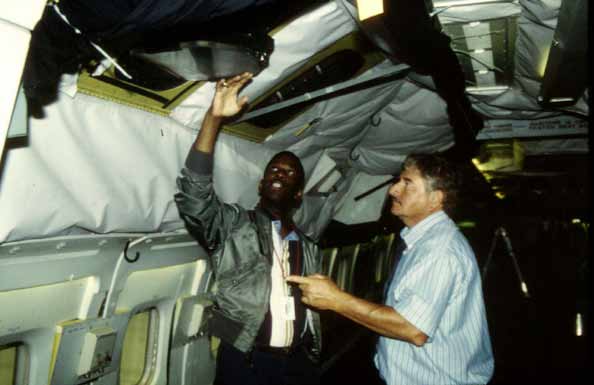
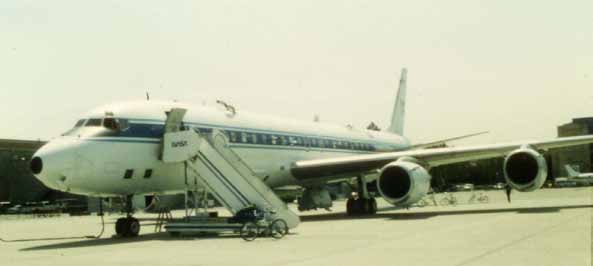
Not all aircraft can carry large numbers of instruments or people, but some can be quite important based on their flight capabilities, such as being able to reach very high altitudes. In the case of the ER-2, where space is very limited, automated and miniaturized sensors are used while taking advantage of primary benefit of using aircraft: the ability to control where they fly. This allows specific altitudes and locations of the atmosphere to be studied. All of these benefits come at a cost—research with aircraft is quite expensive, and, long term, continuous collection of data is not possible. Aircraft are most useful for studying the detailed chemical reactions over a relatively brief period of time.
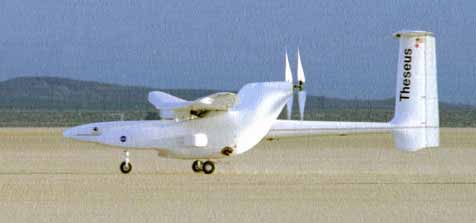
A person is in the cockpit of the ER-2. This aircraft files at very high altitudes, but it can only take one person along—the pilot.
(Photo by Cary Sneider.)
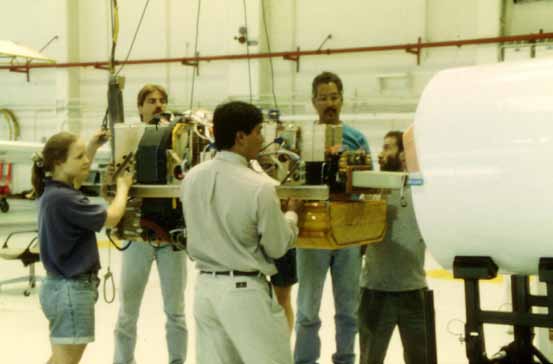
(Photo by Cary Sneider.)
Technology is being advanced to create Unmanned Aerial Vehicles that can carry instruments weighing hundreds of pounds to altitudes exceeding 27 km for several days at a time. These aircraft will be quite valuable for future data collection because pilot/crew safety is not an issue, the aircraft and instruments are recoverable, and the flight path and altitude controllable.
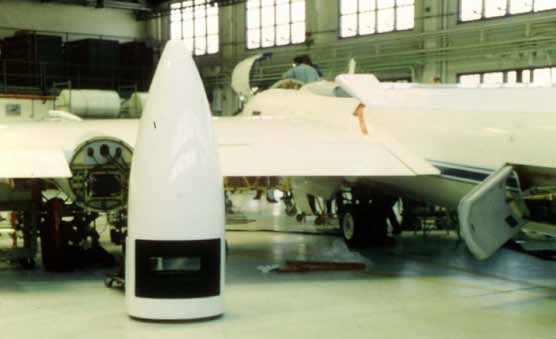
The Theseus, an Unmanned Aerial Vehicle, can fly instruments to high altitudes for several days at a time.
(Photo courtesy of the NASA Dryden Flight Research Center.)
Rockets
By the early 1960s sounding rockets were used to measure profiles of ozone levels from the ground to an altitude of 75 km. Many countries have their own rocket programs, some starting in the 1960s. A wide variety of rockets have been developed to carry instruments of various weights to specific altitudes. Rockets can be flown in most weather conditions, and costs can be lowered if surplus rocket engines are available from the military. Rockets do not fly on a daily or weekly basis because it often takes a minimum of six months to build the instruments and rockets. Teams of researchers and technical support staff are needed to develop the sensors and rocket, launch and record the data, and recover the rocket and sensor. While rockets can go very high in the atmosphere, they can collect data over a small area for a very limited period of time.
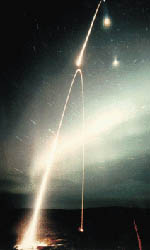
Rocketsonde launch from NASA’s Sounding Rocket Program at Wallops Flight Facility.
(Photo courtesy of NASA.)
III. Satellites
Ever since the Soviet Union launched the Sputnik satellite in 1957, scientists realized that vast regions of Earth’s atmosphere and surface could be measured by instruments in orbit. NASA’s first Earth observing satellite, TIROS-1 (Television Infrared Observation Satellite), was launched on April 1, 1960. The purpose of TIROS-1 was to improve weather forecasting with pictures from space. Two onboard television sensors stored images onto magnetic tape for later broadcast to receivers on the ground. Although TIROS-1 lasted only 78 days, it proved to be a huge success in providing valuable information about the Earth and its atmosphere, regardless of the weather conditions on the ground.
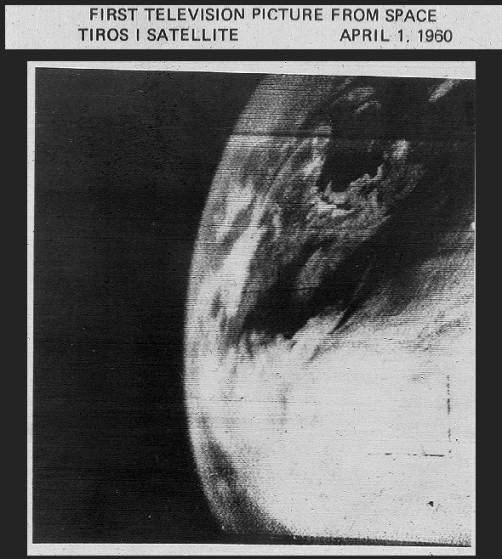
Planet Earth from TIROS 1: First TV Image
(Photo courtesy of TIROS Program, NASA)
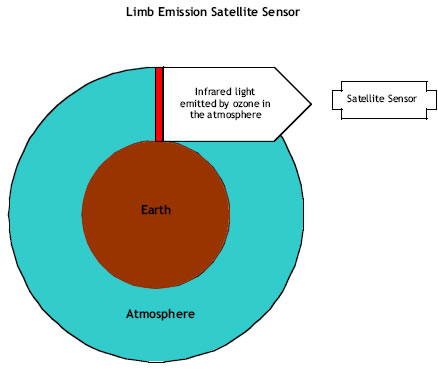
By late 1978, satellites were measuring ozone concentrations over the entire globe. The first satellite that carried ozone sensors was NIMBUS-7. It carried three ozone sensors: LIMS, SBUV and TOMS.
LIMS was designed to measure ozone concentration at different altitudes by measuring the intensity of infrared (IR) radiation emitted by the ozone as observed edgewise at the atmosphere, looking sideways toward the Earth. This technique is called limb scanning. LIMS also measured water vapor, NO2, HNO3, and temperature.
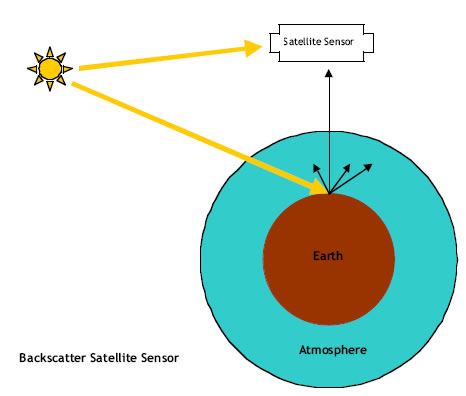
The SBUV and TOMS instruments measured ozone concentrations using the same principle described in Chapter 5: compare the spectrum of the incoming solar radiation to the spectrum of the radiation reflected and scattered (referred to as backscatter) from the atmosphere and the Earth’s surface.
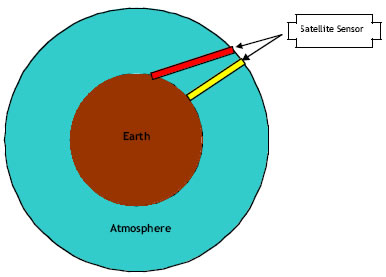
TOMS mapped global total ozone daily by scanning the sensor back and forth beneath the satellite’s orbital track. The accuracy of TOMS total column ozone measurements is estimated to be ± 5%.
It was the TOMS data that NASA reanalyzed to confirm the local ozone depletion observed by the British and Japanese ground stations during the mid 1980s. The data also showed the size of the region experiencing ozone depletion. NIMBUS-7 lasted longer than any other Earth observing satellite, providing data until mid-1993. TOMS has provided almost continuous, global coverage of total column ozone measurements since 1978 until today by being flown on later satellite platforms.
History of ozone measuring satellites:
https://rammb.cira.colostate.edu/dev/hillger/ozone-monitoring.htm
Space Shuttle
Similar to aircraft, NASA’s space shuttle can carry a large number of instruments to high altitudes along with people to operate the equipment. Since 1989, the Shuttle has periodically conducted ozone experiments using a backscatter-measuring instrument. Because of the unique abilities of the shuttle, the instrument is carefully calibrated before launch, during flight, and after landing, as well as controlling where measurements are made. These controls allow ozone data to be correlated between the TOMS and other satellite ozone measurements. These frequent flights are used to ensure the accuracy of ozone measurements from satellites.
NASA Ozone Watch page:
https://ozonewatch.gsfc.nasa.gov
Ozone Monitoring Instrument (OMI) on NASA’s Aura Mission satellite:
https://aura.gsfc.nasa.gov/omi.html
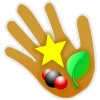
OZ8.1. Investigation: Ozone Measuring
There are many ways to measure ozone in the atmosphere, and each technique has its strengths and its limitations. Discuss the various techniques you have read about in this chapter. Make a concept map showing differences in how the instruments measure ozone, taking into account such things as vantage point, use of optical or chemical sensing, types of sensors (UV, chemical reaction, etc.), how long it can measure the data (e.g. seconds versus days), extent of coverage; reliability, cost, efficiency, complexity of the equipment, and number of people needed to complete the observation mission. You may opt to use some of the quality web sites listed in the appendix of this book dedicated to the technology of these research efforts.

Resolution of Satellite Data
What does resolution mean? Resolution is the scale of the detail that can be observed by the sensor. Have you noticed that the world seems different when looking out of a tall building or looking down from an airplane compared to when you are kneeling on the ground?
You cannot see features that have a size smaller than the resolution of the sensor, or in the case above, your eyes. As much as we would like to be a super hero, our eyes do not have infinite resolution—there is a limit to the size of things we can see. For example, if your eyes have a resolution of 0.001 cm for an object 2 meters away, then you will not be able to see details of an object that have diameters less than 0.001 cm. A pixel represents the average of all the information that is smaller its size.
Examine the details in Picture A (below), which is made up with 262,144 “blocks” or pixels.
If you could see the same flower picture from the same distance as before but with one-forth the number of pixels (16,384), the picture would look like Picture B (below).
Can you see the same level of detail in both pictures? How would this picture look if you could see even fewer pixels? The next two pictures (C and D) are of the same flower, each made with fewer but larger pixels. Picture C is made of up 1,024 pixels, and Picture D has only 64 pixels. Can you even tell you are looking at the same image let alone the inside parts of a flower?
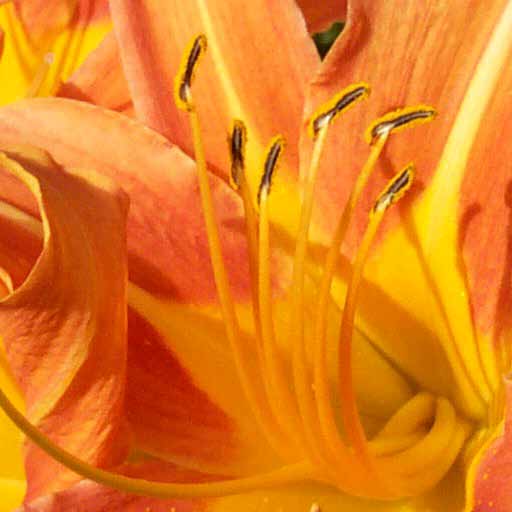
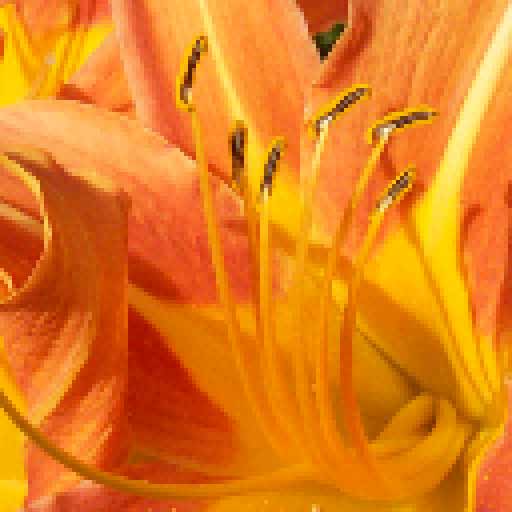
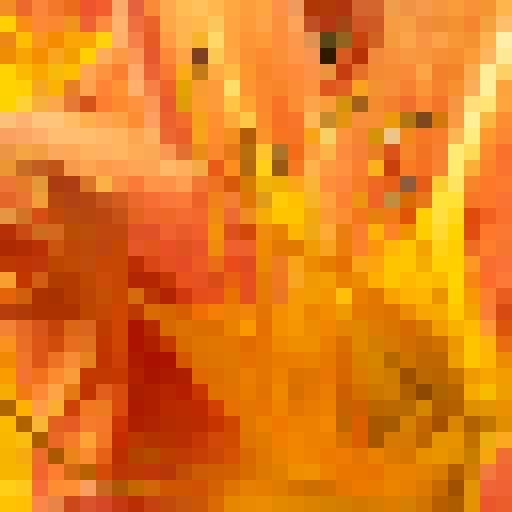
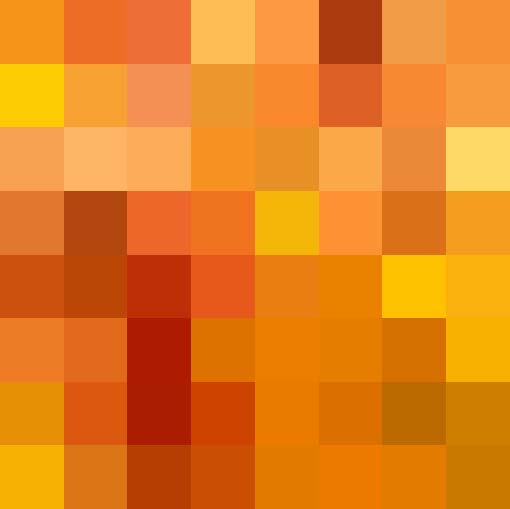
Each of the pixels that makes up a satellite image has the same color across the entire area. You could think of a pixel as a tile or dot of a solid color. The larger the pixel, the less detail within the image that you can see since the details get averaged into the color of the larger tile. Also, larger pixels are more noticeable than smaller pixels. Look at a computer monitor or television screen with a magnifying glass. Can you see the pixels of color? From a distance, the pictures on a computer or television appear smooth, but up close, you can see the pixels.
The example on these two pages dealt with horizontal resolution—pixel size in the east/west and north/south directions. However, ozone concentration changes with altitude as well as horizontally. How well does a satellite sensor “see” these details? When discussing vertical resolution, think of layers, such as that of a birthday cake. This birthday cake is very special—each layer is a different flavor from all the others, but the baker will only make the cake a certain height so it can fit into the oven. The thicker the layers, the fewer the flavors making up the cake. Similarly, if the satellite sensor has a coarse vertical resolution (it can see only a few layers), the fewer details, or “flavors”, of ozone that can be observed.
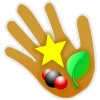
OZ8.2. Investigation: Pixels
Open the DigitalImageBasics software and make sure the Pixel button is active. Open any jpeg format image (e.g. from the Worldwide Web or a digital camera) and see what happens when you reduce the resolution.