EF5. What Is Light?
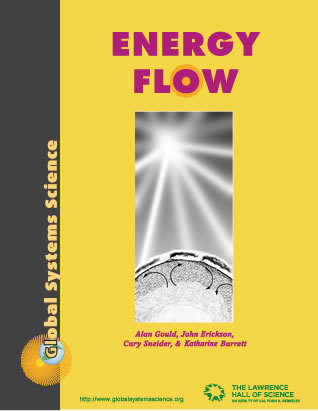
Chapter 5
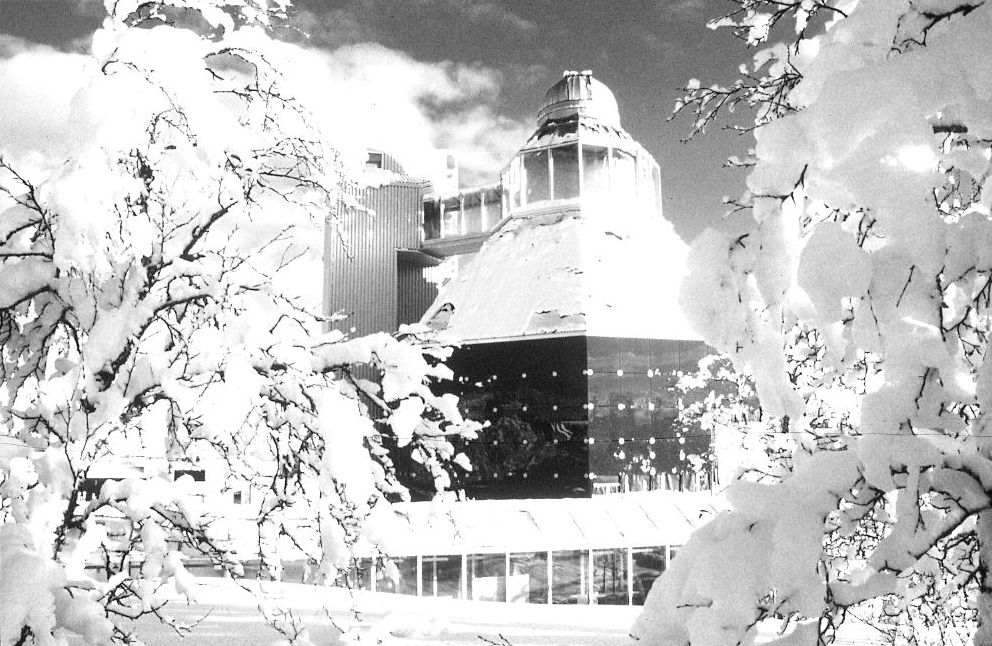
Photograph courtesy of Franck Petersson.
It is 10°C below zero outdoors, and the snow is piled high against the walls of the Tromsø Planetarium—the most northerly planetarium in the world.
But it’s warm and cozy indoors, where Planetarium Director Franck Petersson shows us some of his most recent photographs of the aurora borealis, or northern lights. Tromsø, Norway, where Franck lives with his wife, Marit, who is also a teacher, is nearly 400 kilometers above the Arctic Circle.
“The aurora,” explains Frank, “is evidence that the Earth’s magnetic field is protecting us from one form of Solar radiation that would be quite dangerous to us—the solar wind.
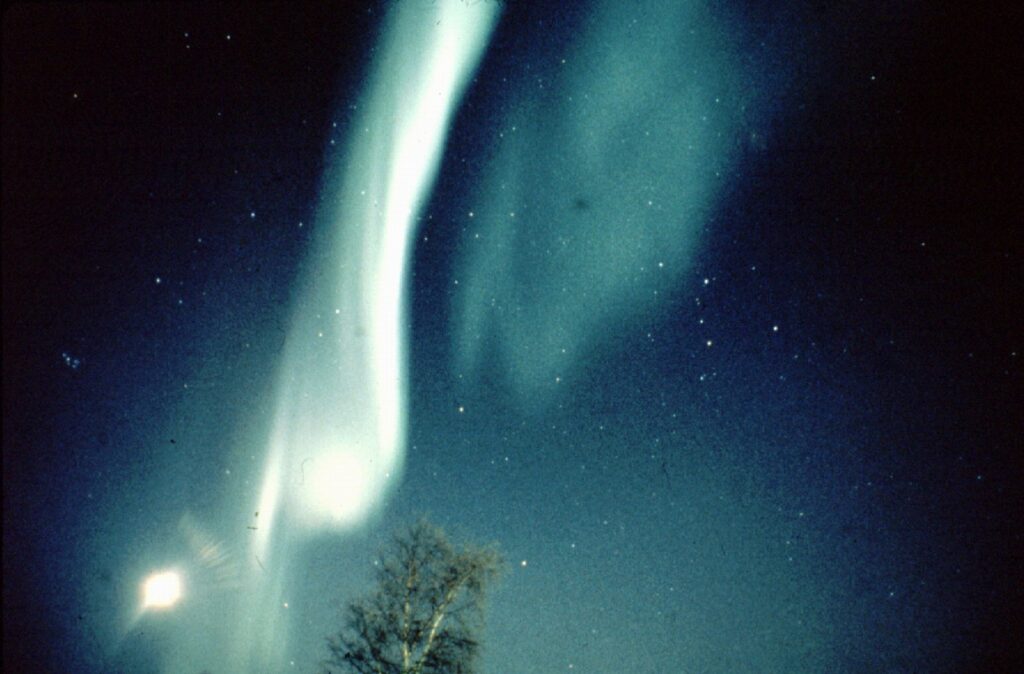
The solar wind is actually part of the corona, the outer atmosphere of the Sun. It is made of electrically charged particles—electrons and protons—that continuously stream outward from the Sun. The Earth’s magnetic field, that allows our compasses to work, channels the particles to the North and South Poles of the Earth. Where the particles strike the upper atmosphere, they strike molecules of air, which is mostly nitrogen and oxygen.
The air molecules absorb the energy from the solar wind, and then release that energy in the form of visible light photons—which we see as the lovely colors of the aurora.”
“Of course,” he adds, “most of the Sun’s radiation is in the form of photons—packets of energy with no mass and no electric charge. The photons penetrate the magnetic field and reach the Earth’s atmosphere, where they give us heat and light….except”—he adds with a shudder—“above the Arctic Circle in the winter!”
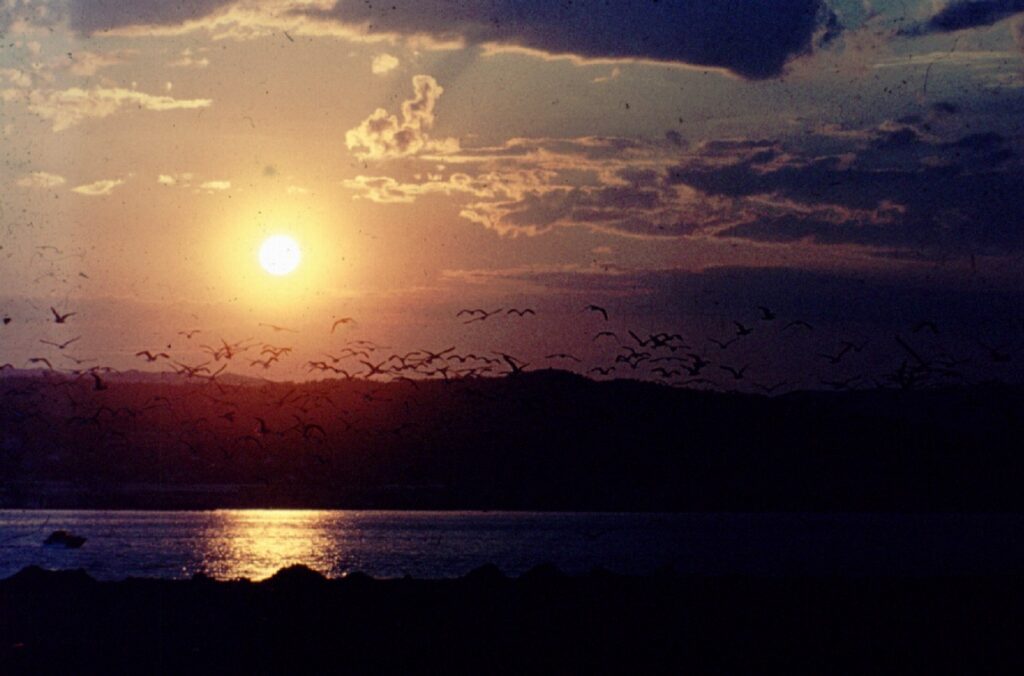
That reminds Franck of his pictures of the midnight sun. “That’s when the Sun remains in the sky night and day for several months. It never gets very high in the sky, but it’s never completely dark, either,” he explains. “In the winter, it’s just the reverse. We don’t see the Sun at all for several months.”
He shakes his head and tells us that, “The long night is definitely the worst. Even people who are born here never get used to months of darkness; and that’s why I’m especially interested in the lovely, colorful streamers that frequently appear in the winter sky.”
I. What Is Light?
In the last chapter, we learned of the source of the Sun’s light. This is the ultimate source of most of the energy flowing around us, including the constant stream of electric signals triggered by photons of light hitting nerves in the retinas of our eyes. Light fills our world—we see everything in our homes, admire trees and flowers, read books and maps, find our way to school or work, watch movies and concerts, marvel at the spectacle of aurora borealis. But what is light?
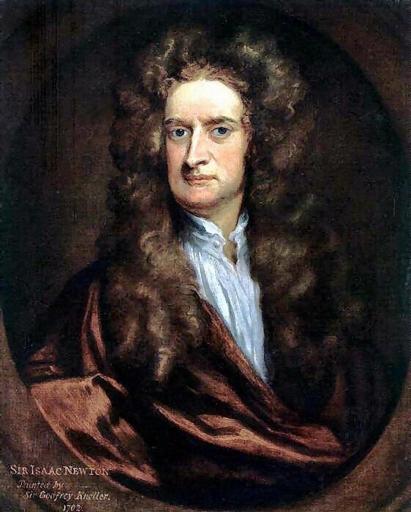
Isaac Newton favored the idea that sunlight was composed of streams of tiny particles. Particles of light are called photons. He thought that there were different colors of light particles—blue light being composed of blue particles, red light composed of red particles, and so forth. Other investigators thought light acted more like a wave than a particle. For the wave proponents, color was determined by the length of the wave, from crest to crest. Blue light had a shorter wavelength than red light, and each of the colors of the visible spectrum had its own specific wavelength.
Many experiments indicated that light was a wave motion rather than a stream of particles. But it was a mystery as to what was vibrating! Water waves travel through water. Earthquake waves travel through rock and soil. But light moves through the almost-empty space between the Sun and Earth. Even before it was established that there was no air between the Earth and the Sun, experimenters had removed air from glass jars and found that light readily passed through. The answer to what was waving came from experiments with electricity and magnets by scientists such as Michael Faraday.
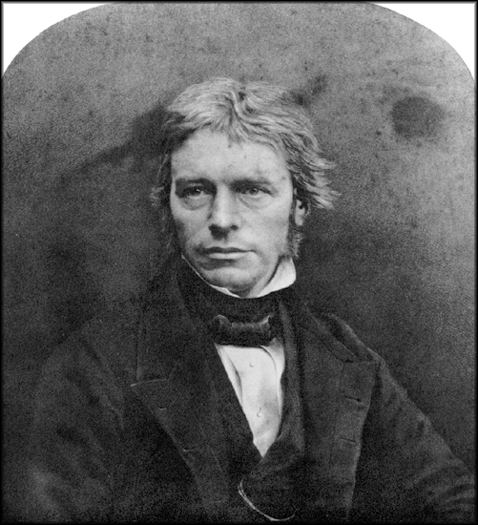
http://siarchives.si.edu/history/jhp/papers01.htm
Michael Faraday, the son of an English blacksmith, made a series of discoveries that would change the world. Faraday began his work in science as a washer of laboratory glassware. Later he became the head of the laboratory. In 1821 he began a series of experiments that were to introduce the age of electricity.
Only the year before, Danish physicist Hans Christian Oersted had discovered that electricity flowing in a wire produces a magnetic field around the wire.
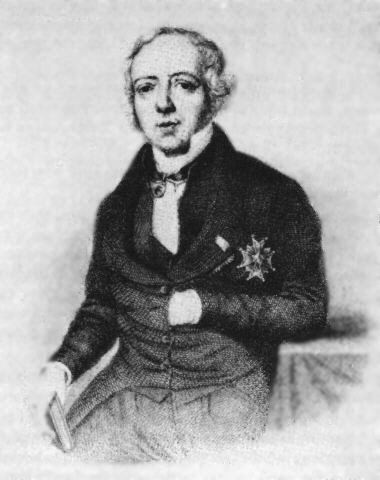
http://www.th.physik.uni-frankfurt.de/~jr/gif/phys/oersted.jpg
Faraday built on Oersted’s discovery and found that the converse also works: a moving magnet produces an electrical current in a wire. That discovery eventually led to the invention of electric generators, which now produce most of our electric current. Faraday’s work also laid the foundation for the development of electric motors.
On a spring evening in 1846 Michael Faraday was to introduce another scientist as the speaker of the evening at a lecture for people interested in science. At the last moment the speaker got cold feet and left the meeting. Faraday was left standing alone on the platform. In order not to disappoint the guests he explained his work with moving magnets and the production of electricity. First, he explained that a magnet had an invisible magnetic field around it. He could make the field visible by holding a magnet under a sheet of paper, and sprinkling iron filings on the paper. The pattern of the iron filings revealed the presence of the magnetic field. It was this field which attracted iron objects that came within its range.
Faraday wondered what happens to the magnetic field of a magnet if you move the magnet up and down rapidly. If you shake the end of a rope up and down, the rest of the rope responds by moving up and down and the disturbance moves down the rope as a wave. Faraday thought that surely the movement of a magnet would cause its magnetic field to move as well. He knew that a moving magnet could produce an electric current in a nearby wire. Might the disturbance in the magnetic field move out beyond the wire? Possibly, he reasoned, moving the magnet up and down produced a moving wave in the magnetic field; and just possibly, that is the way that light might travel.
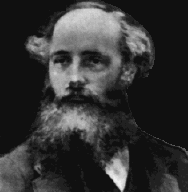
http://imagers.gsfc.nasa.gov/ems/
consider.html
A young Scottish mathematician named James Clerk Maxwell heard about Faraday’s ideas. He knew that magnets are surrounded by magnetic fields and that there are also electric fields that surround objects that have static electric charge. Your most direct sensation of these electric fields has probably been the feel of “static cling” of certain fabrics, especially when they are fresh out of a dryer after having been tossed about and rubbed against other fabrics producing an excess or deficiency of electric charge on some of them. You may also have experienced the result of electric field if you felt a shock from touching a door knob or other metal object after you accumulated enough electric charge when the soles of your shoes rubbed a carpet as you walk. Electric fields attract (or repel) charged particles such as electrons and move electrons in wires, making electric current.
Maxwell asked himself: if electricity flowing in a wire could cause a magnetic field, and a moving magnetic field could cause an electric field that makes electricity flow in a wire, what was the relationship between the two fields? How fast could a magnetic field produce an electric one and how fast could the electric field produce a magnetic one?
Maxwell had the mathematical skill to work out equations that allowed him to determine how quickly such changes would occur. When he found the answer he was astounded. The speed of propagation of such changes was the same as the speed of light. A theory immediately suggested itself to him and later experiments indeed proved that light is a wave of changing magnetic and electric fields that travel through space. In other experiments, light acts as if it were made of particles, so we are forced to accept this dual nature of light—it acts if it were both waves and particles.
II. The Electromagnetic Spectrum
What do you think of when you hear the word “radiation?” Danger? Nuclear disasters? Mutant creatures with super powers? The word “radiation” is used so many ways that it is easy to get different kinds of radiation mixed up. In general, the word “radiation” can apply to anything that moves outward from some central point. In this sense, a “boom box” speaker is the source of “sound radiation,” but it is not dangerous to you, unless the music that is radiating is dangerously loud or offends someone with violent tendencies.
Energy from the Sun is generally called electromagnetic radiation. As described previously, it is composed of oscillating electric and magnetic fields.
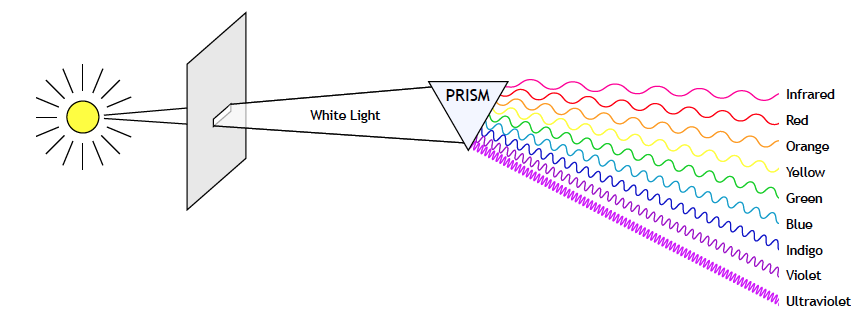
Radiation transfers happen in packets of energy—normally miniscule amounts of energy—called photons. Many forms of electromagnetic radiation are probably familiar to you. Light is one form of electromagnetic radiation. Others include infrared, microwave, radar, television and radio waves. All of these have wavelengths longer than that of visible light. Other kinds of photons, with shorter wavelengths than light, are ultraviolet, x-rays, and gamma rays. The full range of these vibrations is called the electromagnetic spectrum.
Long wave radiation. The longer the wavelength, the less energy in each photon. Here are types of photons with wavelengths that are longer than visible light:
Radio waves are the longest electromagnetic photons known. Although they are the least energetic, they can carry messages through clouds and across space.
Microwaves are shorter and more energetic than radio waves. Microwaves are used in ovens to heat food, and for communications.
Infrared radiation (abbreviated IR). All objects give off IR radiation to some extent; hotter objects give off more infrared radiation than cooler ones.
Visible light radiation. The photons that we can sense with our eyes are in the middle of the electromagnetic spectrum. Photons of red light have less energy than photons of violet light.
Short wave radiation. The shorter the wavelength, the more energy in each photon. Here are the types of photons with wavelengths that are shorter than visible light:
Ultraviolet radiation (abbreviated UV) has higher energy than violet light. It is sometimes referred to as ultraviolet light, but it is not visible to humans. (Honey bees can see ultraviolet light.)
X-rays have even more energy than UV. No one outside the comic books has eyes that can see x-rays but these invisible rays can be used to take pictures. Since x-rays can shine right through many solid objects, pictures made with x-rays are used to reveal the insides of people and airline baggage.
Gamma rays include all electromagnetic radiation with more energy than x-rays. Gamma rays are produced by nuclear reactions deep inside the Earth, in the Sun, and in other stars.
III. Our Atmospheric Shield
The most energetic photons produced by the Sun are dangerous for people and other living things. Large doses of gamma rays can kill rather quickly. X-rays were once thought to be harmless, and shoe stores even installed X-ray machines so people could watch their toes wiggle inside their new shoes. The machines were removed when it was discovered that in time, X-rays can cause cancer. Even today, X-ray technicians wear lead aprons to protect themselves, and dosages are kept as low as possible for patients who must have X-rays taken of their bones and teeth.
Ultraviolet radiation can also be dangerous in large doses. It can kill microorganisms quickly. Ultraviolet photons cause sunburn, and it is likely that damage caused by UV is cumulative throughout a person’s lifetime, and can lead to skin cancer. UV is also blamed for cataracts—a condition in which the clear covering of the eyes becomes cloudy and can lead to blindness if not treated with surgery. Animals do not wear hats and sunglasses, so they cannot protect themselves from UV radiation.
Fortunately we are shaded from gamma rays, X-rays, and most ultraviolet radiation by the layer of ozone gas high in the atmosphere. There is serious concern about depletion of the ozone layer due to the use of certain human made chemicals, called chlorofluorocarbons, or CFC’s.
Other forms of electromagnetic radiation are generally considered safe. However, any form of electromagnetic radiation, if made intense enough, can cause heating and burning. Think of what happens when you use a simple magnifying glass to focus the Sun’s rays!
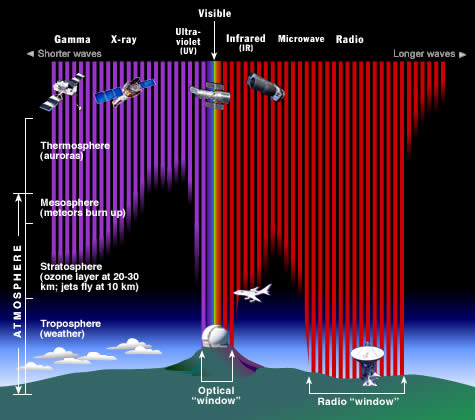
This diagram shows what happens to energy across the electromagnetic spectrum when it encounters the Earth’s atmosphere.
Notice that most UV photons are absorbed in the stratosphere, where the concentration of ozone is highest. X-rays and gamma rays are also absorbed in the atmosphere. Some IR is absorbed as well, due to water vapor, but much of it gets through. The fact that visible light easily penetrates the atmosphere is probably responsible for the fact that complex life forms on Earth have evolved eyes that can sense visible light.
Question 5.1
How many things at your home or in other places you go depend on types of radiation other than visible light? Some examples are given on this page. Make a list of as many things as you can and explain what type of radiation is involved.
IV. Conclusion
This short chapter presents a very important net of ideas that you will need to understand energy flow through the atmosphere—that light is a form of energy that acts as both a wave and a particle. The next chapter focuses on what happens to infrared photons in the Earth’s atmosphere. The fate of those photons has great bearing on the average temperature of the Earth.