EF3. What Heats the Earth’s Interior?
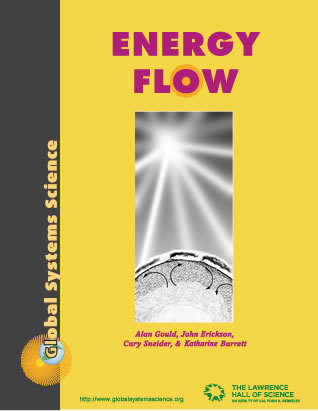
Chapter 3
The convection currents discussed in the last chapter explain most of the world’s volcanoes, because most volcanoes occur along the edges of plates. In some of these places, the rift zones, plates are spreading apart, so magma comes up from below to cause the volcanoes. In other cases, the subduction zones, one plate is diving under another. When it melts, it causes volcanoes near the edge of the upper plate. Mount St. Helens erupted in a subduction zone.
But the volcanoes of Hawaii do not fit either of these patterns. The Hawaiian chain of islands is a series of huge volcanic peaks, poking up above the surface of the ocean, right in the middle of the Pacific Plate! Earth systems scientists explain the Hawaiian Islands by assuming that a “hot spot” exists in the mantle under the plate. That is a region in which the magma is hotter than the surrounding magma, so it naturally rises and punctures the relatively thin oceanic plate above it.
Very good evidence for this explanation is the shape of the Hawaiian Island chain. The islands form a long line, with the youngest islands on one end and the older islands on the other. The very youngest is the Big Island of Hawaii which is being formed even today. The line of volcanoes occurs as the Pacific Plate moves slowly over the hot spot. The very active Kilauea Volcano continues to erupt, sending streams of lava into the ocean, because it is directly over the hot spot.
In recent years the discovery and mapping of vast lava flows throughout the world has suggested a new theory. In addition to convection currents in the upper mantle and crust, another process is at work to release the energy from deep inside the Earth. This idea is illustrated in the cut-away view of the Earth on the next page. It shows the formation of huge plumes of hot magma at the boundary between the Earth’s outer core and the mantle.
The Earth’s outer core is composed of molten metal. It is denser than the rocky mantle, and it is also hotter. The core heats the lower mantle by conduction. When a blob of magma, or plume, becomes hot enough, it expands and floats to the surface. A small plume creates a “hot spot,” like the one under Hawaii. When a huge “superplume” reaches the surface, it can create dozens of volcanoes and massive lava flows over vast areas.
I. Why Does Hot Magma Rise?
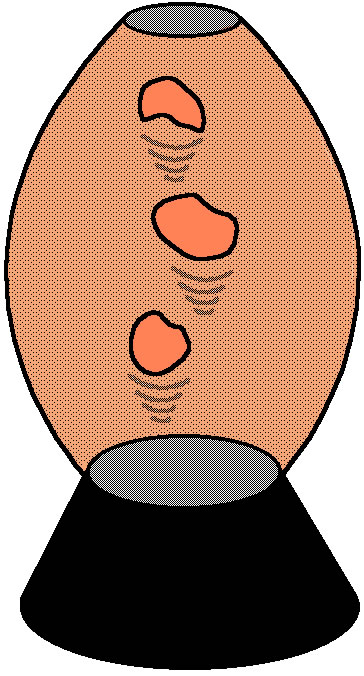
Long ago, people thought that heat was some kind of fluid. They even gave it a name—“caloric.” Something that was warmer was thought to have more caloric in it than something that was colder. The caloric theory of heat explained why a pot of water heated up when held over a flame—caloric flowed from the hot flame into the cold liquid. However, this theory could not explain how energy of motion could be converted to heat; nor could it explain why heating made a liquid or gas rise. So, it was abandoned by scientists more than a hundred years ago, in favor of a more useful theory, the kinetic theory of heat.
The kinetic theory of heat used by scientists today is one aspect of the particulate theory of matter—the idea that all matter is made up of tiny particles.
In the particulate theory, all materials are made of basic building blocks called atoms. Materials made of just one kind of atom are called elements. The natural elements in the universe include carbon, oxygen, hydrogen, gold, copper, uranium, and 86 others (total of 92 natural elements). There are also about 25 man-made elements, most of which do not survive very long without breaking down into one or more of the natural elements. Everything else is made of clusters of atoms called molecules. For example, water molecules each contain two atoms of hydrogen (H) and one atom of oxygen (O). A molecule of water is abbreviated H2O.
In this theory, whether something is a solid, liquid, or gas depends on the relationships among its molecules. If the molecules are locked together rigidly, the substance is a solid. If the molecules can slide by each other so that the substance can flow to take the shape of the container, it is a liquid. If the molecules are far apart and can spread out even further to fill the container completely, the substance is a gas.
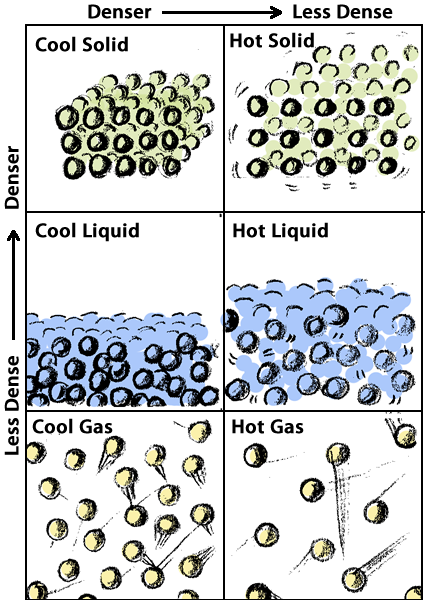
Heat in Solids – According to the particulate theory of matter, atoms and molecules are always in motion. Even in solids, the atoms and molecules vibrate. If energy is added to the solids, the molecules vibrate even more. If we touch the solid when its molecules are vibrating faster, we sense this movement as heat.
Heat in liquids – In we add enough energy, the molecules may vibrate fast enough to break the bonds that hold them in a rigid structure, and the substance will melt–the molecules will flow past each other and the substance will become a liquid.
Heat in gases – If we continue to add more energy, the molecules will move faster. With enough energy, the molecules will move so fast that they break the bonds that hold them to other molecules completely and the substance becomes a gas, expanding to fill the container.
According to the particulate theory of matter, heat is the motion of molecules. Adding energy to a solid can cause its molecules to vibrate faster and faster until they break free of each other and flow past one another. We observe that change from solid to liquid as melting. Add more energy and some molecules will move so fast that they can escape from their neighbors entirely. We call that change from liquid to gas, evaporation or vaporization.
Deep inside the Earth, molecules of rock are moving so rapidly that they slide past each other and flow. The hot rock becomes a viscous liquid—magma. The magma is heated more in some regions than in others. Additional heating causes the molecules to move faster and spread out more. In those regions the magma becomes less dense.
Cooler, denser magma has a stronger gravitational attraction to the rest of the Earth, so it gets underneath and pushes up the less dense magma. That is why hot magma rises to the surface and erupts through rifts. When it reaches the surface it cools, solidifies, and becomes denser. Eventually, it sinks and it is melted again inside the Earth. The convection current continues, driven by differences in density.
II. Understanding Density
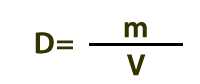
Density is defined by this equation. If says that density (D) is the amount of matter (m) in a given volume (V). As shown in the illustrations in the previous section, cooler materials are generally denser than warmer materials; and solids are usually denser than liquids, which are usually denser than gases.
III. What is the Source of the Earth’s Heat?
Throw a handful of oatmeal into a pot of water, put it on a hotplate, and watch it boil. When it heats up, you’ll see one or more convection currents. The action will be much faster than the movement of magma within the Earth, but convection in the pot functions the same way. It transports heat from the bottom of the pot to the top, and releases it into the air.
Every convection current requires a source of energy. For the boiling oatmeal, the source is the hotplate, which in turn gets energy through a wire from your local power plant. The source of the energy that drives convection currents inside the Earth comes a tiny bit from left over heat from Earth’s formation.
Heat from gravitational collapse
Although most of the heat in the Earth comes from radioactive decay, some of the Earth’s internal heat is due to gravitational energy left over from when the Earth first condensed from a cloud of gas and dust, about 4.5 billion years ago. At that time, gravitational forces caused all the pieces of the solar system to fall gradually inwards towards the place where the Sun ultimately formed. The increasing speeds of the particles and gases led to overall heating of the system.
Below is Isaac Newton’s Universal Law of Gravitation. The gravitational force, which depends on the masses of the objects (m1 and m2) as well as their separation (r), leads to increasing speeds of the masses, hence heating.
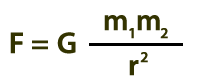
F = gravitational force
m1 = mass of object 1
m2 = mass of object 2
r = separation of masses
G = universal gravitation constant
Although a small amount of the energy that drives convection currents inside the Earth comes from left over heat from Earth’s formation, most of the heat in the Earth is from radioactive decay in which the center, or nucleus of an atom emits a tiny particle, resulting in the atom changing into a different kind of atom, and surrounding atoms getting heated up.
An atomic nucleus contains two kinds of particles—protons and neutrons. The number of protons gives an atom its identity. For example, each hydrogen atom has just one proton, helium has two, and carbon has six. The protons have positive electrical charges which tend to repel each other and split the nucleus apart. Neutrons have no electrical charge. They provide some of the strong attractive nuclear force which holds the nucleus together.
Larger atoms are unstable. Uranium has the largest number of protons of any naturally-occurring element—92. It also has 146 neutrons, which help to hold the nucleus together. Uranium is unstable. On average, it takes about 4.5 billion years for half of the atoms in a sample of rock to decay and become stable lead. Since the Earth is about 4.5 billion years old, about half of the uranium in the Earth has become lead.
When an atom of uranium or some other radioactive material splits, it releases a small amount of energy. The energy is radiated from the atom in the form of alpha particles, beta particles, and gamma rays. That means the energy travels through space. But inside the Earth there is not much space at all! The energy is quickly absorbed by a molecule of rock or metal, causing it to heat up.
Although each atom gives up only a little energy when it decays, the huge number of atoms that decay every second keep the interior of the Earth at a very high temperature. Radioactive decay is the major source of heat that energized the eruption of Mount St. Helens, and every other volcano and earthquake on our planet.
Some of the heat energy comes to the Earth’s surface by conduction and convection. This heat from deep in the Earth is an energy source that drives huge convection currents in the ocean. Water from the polar ocean regions is very cold and dense. It sinks and displaces the bottom layer of water which has been warmed by heat from the Earth’s interior. The warm water is pushed upward towards the ocean surface.
IV. Superplumes and Earth’s History
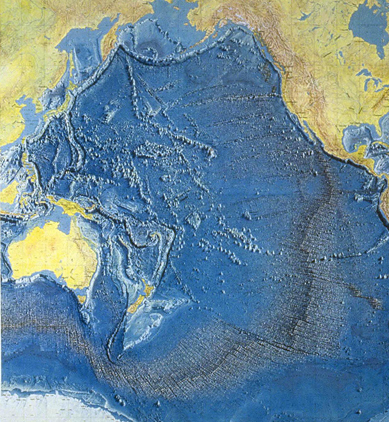
On this picture of the floor of the Pacific Ocean, observe the vast difference in terrain of the eastern and western sides of the Pacific. The eastern side is a smooth, striped pattern, while the western side is a chaotic jumble of volcanic mountains and plains. The terrain reflects the two different ways that these sides of the ocean floor were formed.
The eastern Pacific floor was formed by the orderly process of convection in the upper mantle and crust as described earlier in this chapter. The western Pacific puzzled scientists until recently.
One clue came from deep ocean drilling, which allowed scientists to take samples of rock from different depths. They found that the bottom layers contained tiny fossils that dated from the Jurassic Period, which lasted from 208 million years ago to 144 million years ago. On top of that were thick deposits of lava from the Middle Cretaceous Period, about 120 million years ago.
The evidence very clearly points to a massive period of volcanic activity over a relatively “short” period of just a few million years. The level of activity must have been immense—on the order of ten Mount St. Helens eruptions every month, for thousands of centuries. Many scientists now believe that the volcanic activity was due to a superplume that reached the top of the upper mantle, causing great rifts in the crust and hundreds of volcanoes. The floor of the western Pacific still shows those scars.
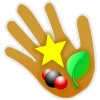
EF3.1. Investigation:
Observing Convection Currents
This is a simulation to visualize the process of convection currents beneath the Earth’s crust. [Note: this is the same investigation as in GSS Life and Climate chapter 7.]
V. Volcanic Influence on Atmosphere and Climate
The effect of the volcanic activity on the Earth’s climate history was profound. The Middle Cretaceous Period was the warmest of any time before or since. Fossils of that period from all over the globe show that much of the planet was covered with tropical forests. Dinosaurs roamed the continents, and the seas teemed with life.
The connection between the volcanoes and the Earth’s climate is not simple. Some warmth came from the volcanoes themselves, as they released heat from inside the Earth, but those effects were local and short-lived, like the eruption of Mount St. Helens. It is more likely that the worldwide climate change was due to vast quantities of carbon dioxide that the volcanoes released into the atmosphere.
Carbon dioxide is a “greenhouse gas.” It traps heat in the atmosphere, making the Earth warmer. (More on this in Chapter 7.) Normally, this gas circulates through the Earth system very slowly. Carbon dioxide in the atmosphere dissolves into rain, forming carbonic acid. This natural and very dilute acid rain becomes runoff that dissolves minerals in rocks and these minerals eventually travel to the oceans. There, marine animals take up the carbon dioxide into their bodies, converting it into organic compounds. When they die, their carbon is deposited on the ocean floor in the form of carbonates. After millions of years the ocean floor is pushed deep into the Earth and melted. The melted crust releases the carbon as carbon dioxide gas, which floats to the surface in huge bubbles, along with sulfur and other gases. During volcanic eruptions the gas returns once again to warm the atmosphere.
During a period of high volcanic activity, the part of the cycle that returns carbon dioxide to the atmosphere speeds up. The ocean floor melts faster and huge amounts of carbon dioxide are released, warming the worldwide climate. That’s what happened during the Cretaceous Period.
VI. The Long Term Carbon Cycle
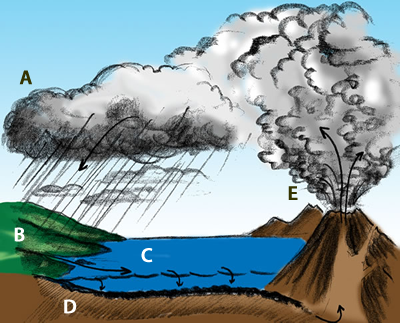
This illustration shows the important role that volcanoes play in cycling carbon through the Earth system. The amount of carbon dioxide in the atmosphere regulates the average global temperature.
The parts of the diagram:
A. Carbon comes out of the atmosphere into plants (and animals that eat plants)
B. Organic carbon from dead life forms is deposited. On land, it forms coal, oil and gas. The organic carbon is pressurized over ages to time to form solid carbon. The coal we burn up in no time took about 300 million years to form.
C. In the ocean phytoplankton absorbs carbon dioxide and in turn is converted by shell forming organisms to calcium carbonite.
D. Through ages of time and pressure in the ocean floor sediment forms limestone and dolomite.
E. Molten rock (lava) reaches Earth’s surface in volcanos that release carbon dioxide.
VII. Conclusion
In this chapter we took a mental journey inside the Earth to find the source of its heat energy and see how it flows to the surface.
For a sense of what’s deeper, see the BBC online interactive
Journey to the Centre of the Earth.
Most of the energy within the Earth begins as radiation and the kinetic energy of decay products from the decay of very large atoms. The energy from radioactive decay does not go very far before it collides with molecules of magma, making them move faster.
The heat is spread throughout a region of magma by conduction, in which molecules hit other molecules, making them go faster too. A large volume of magma heats up and becomes less dense than its surroundings.
While radiation and conduction are important ways that energy flows through the Earth’s interior, most of the Earth’s internal energy escapes through convection. Convection can occur in gradual convection currents in the upper mantle, as described in chapter 2, or in huge plumes of magma that carry hot molten rocks to the upper mantle and erupt through the surface, as described in this chapter (3).