EF6. How Does Energy Flow In the Atmosphere?
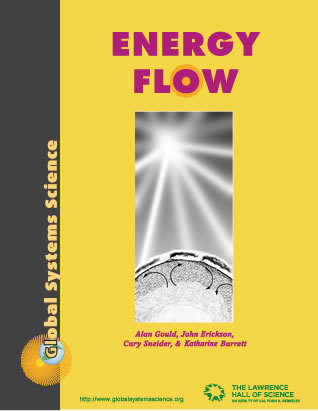
Chapter 6
Most natural systems are continuously changing. Yet within them certain things remain the same, or fluctuate within limits. Any balance between opposing forces or influences can be called “equilibrium.” There are two kinds of equilibrium: static and dynamic.
Systems in Static Equilibrium
Static equilibrium is when parts of a system really are not changing at all. A good example is a rock resting quietly on the ground. It is not changing its position. The force of gravity pulling down on the rock exactly balances the upward force of the ground on the rock. The rock system is in static equilibrium because it is not changing its position at all.
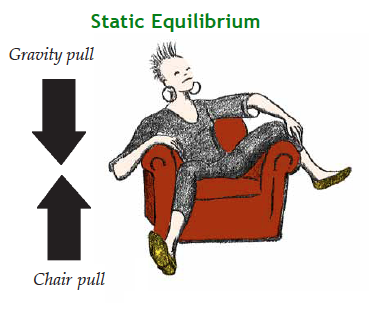
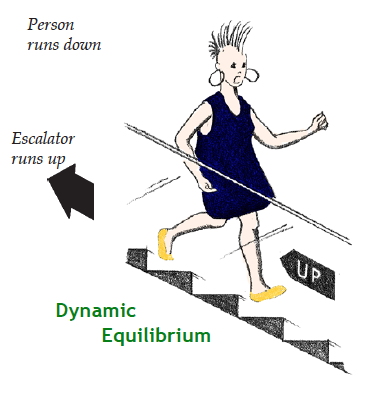
Systems in Dynamic Equilibrium
A system in dynamic equilibrium is always changing, yet certain characteristics of the system stay the same or about the same within limits. A good example is a person walking down an up escalator at just the right rate, so that she stays midway between the ends of the escalator at all times. She is moving and the escalator is moving, but she remains at an equilibrium position in the middle of the escalator. She may not stay exactly at the same spot, but she can maintain her position within a few feet. She can stay in dynamic equilibrium as long as she can keep walking at about the same speed as the escalator is moving. Eventually, however, she will get tired and the system will take her out of the dynamic equilibrium position.
A more complex example of dynamic equilibrium is the total amount of money that a bank holds. The amount of money held in “reserve” remains fairly constant, even though the bank is receiving deposits from people and making loans to other people all the time. Equilibrium can be upset if a lot of people withdraw their savings at the same time. In that case, the flow of money out will exceed the flow of money in, and the cash reserves will be greatly reduced.
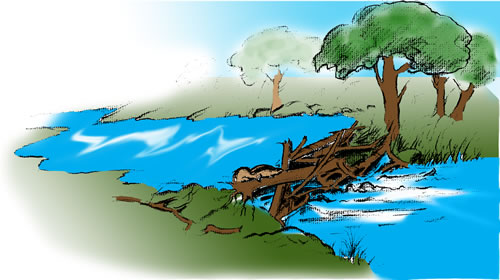
even though water continuously flows into and out of the pond.
The water level in a beaver pond illustrates dynamic equilibrium. A beaver creates the pond by building a dam. Water runs through and over the dam of tree branches. Stream water is continually flowing in and out; while the dam maintains a nearly constant level of water in the pond.
Systems can break down if they are stressed too much or if their maintenance is neglected. A bank can collapse due to major changes in the economy, or failure of the bank officers to make cautious decisions. A beaver dam may be lost if spring flooding is unusually severe, or if the beaver fails to plug holes and maintain the dam.
The Earth-atmosphere system is in dynamic equilibrium since the energy absorbed by the Earth equals the energy it emits into space. The uppermost part of the Earth’s atmosphere is very cold, -18°C; but due to the greenhouse gases, the temperature at the surface is a toasty 15°C. As long as the energy from the Sun remains constant, and the composition of the atmosphere stays the same, the system will remain in dynamic equilibrium, and the average temperature at the surface will stay about the same. But if there is a significant change in the system—such as a change in the Sun’s brightness, or in the composition of the atmosphere, the Earth’s dynamic equilibrium could change, bringing about a global change in climate.
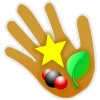
EF6.1. Investigation:
Experimenting With Equilibrium
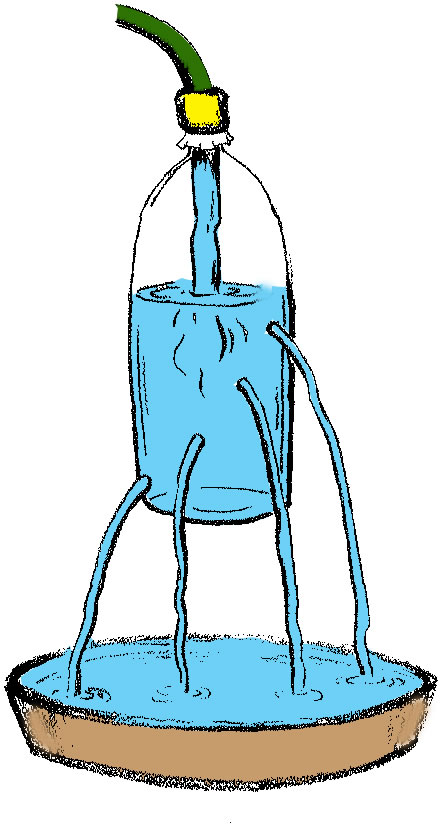
A clear plastic container (such as a 2-liter soda bottle) has a series of holes about 1¦2 cm. in diameter. The holes are evenly spaced from top to bottom, about 2 cm. apart, in a spiral pattern around the bottle. Water flows continuously into the bottle from above, through a tube from a water faucet. Water flows continuously out of the bottle through the holes. A sink or large tub of water catches the water flowing out so it does not spill on the floor.
Predict
- When the water is turned on, what will happen to the level of the water in the bottle?
- What will happen to the water level if one of the holes is blocked?
- What will happen if the water flows into the bottle faster? Slower?
Observe and Record
- Turn on the water and adjust the flow so the water level stays constant. Mark the level with a piece of tape. What happens if you increase (or decrease) the rate of flow?
- Block one or two of the holes. What happens?
Draw Conclusions
- What is the relationship of the water level to the rate of flow?
- What is the relationship of the water level to the number of blocked holes?
- How is the rate of incoming water related to the rate of outgoing water?
- Is this system in equilibrium? Why or why not? If it is in equilibrium, is it in static or dynamic equilibrium?
I. Is Earth’s Climate Changing?
One of the most fascinating questions in global systems science has been whether the Earth is warming up or cooling off. In the 1970’s, the analysis of layers of ice buried near the North and South Poles revealed that for nearly all of the past 160,000 years the Earth has been in the grip of an ice age. Then, about eighteen thousand years ago, it started to warm up. We are currently enjoying a relatively warm period in the history of the Earth, called an “interglacial period”—between glaciers. The long cycle of ice ages is now attributed to very gradual changes in the Earth’s orbit around the Sun, and the direction of its spin axis in space. Researchers predict that in another 5–10 thousand years, Earth would start to cool again.
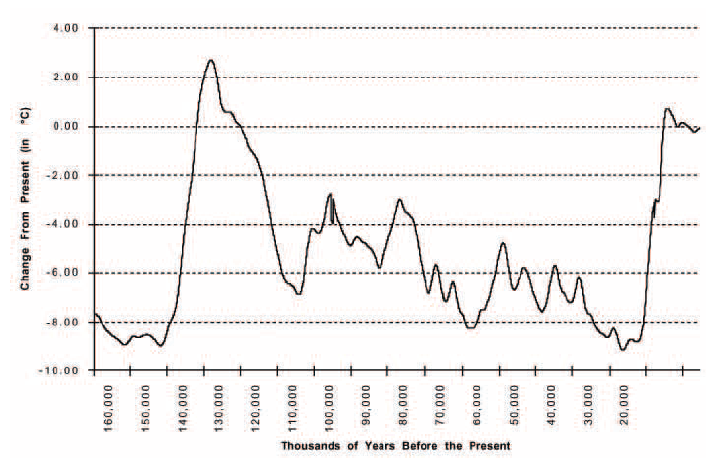
Then, in the 1980’s, a new theory suggested that long before the Earth starts to cool due to its slowly changing orbit, it would warm up—perhaps within 50 or 60 years. The new theory predicted that the Earth would warm because of certain gases that humans are adding to the atmosphere; mostly carbon dioxide from the burning of coal, oil, and natural gas in cars, power plants, and factories. The theory of global warming predicted significant climate changes in a few decades, causing drought in some areas, increased severity of storms in other areas, and a rise in the sea level around the world, which would threaten low-lying areas with flooding.
The issue came to a head in 1988, when climate researcher James E. Hansen of the National Aeronautics and Space Administration (NASA) testified before congress that he was “99 percent certain” that global warming was underway. Since then, the battle has raged between those who agree with Hansen and those who disagree, with new data emerging every few months. The jury is still out on how much the globe will warm and how severe the effects will be; but understanding how solar energy flows through the atmosphere is growing.
The theory of global warming hinges on a process known as the “greenhouse effect.” The experiment on the following pages will help you understand how the greenhouse effect works.
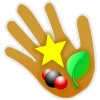
EF6.2. Investigation: Heat Trappers, Inc
Work with a team of engineers to create as warm an environment as possible for some miniature exotic tropical plants for your school’s new botanical garden.
II. How Windows “Capture” Solar Energy
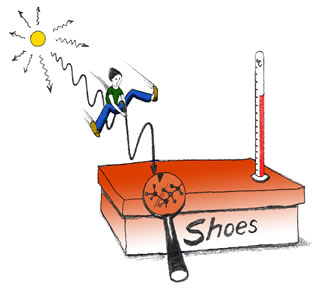
Imagine a photon of visible light winging its way from the Sun. It zooms down through the Earth’s atmosphere and comes in for a crash landing at the speed of light on the cover of the control box (with no windows). The photon encounters a molecule in the box top that absorbs its energy. That vibrating molecule jostles other molecules and causes them to vibrate as well. The box top heats up.
Molecules of air inside the box are bumped by rapidly moving molecules in the box top. So air inside the box gains some heat by conduction through the box top. But most of the Sun’s energy is quickly reradiated back into the space around the box in the form of infrared photons.
If the visible light photon encounters a window in one of the greenhouses, the story is quite different. A window of clear plastic has a transmittance for visible light of 96%. Most photons pass right through to the inside where they crash into the floor of the box and meet molecules that absorb their energy.
The floor of the greenhouse will reradiate infrared photons. Some of these photons move to escape back into space through the window. But wait! The transmittance of plastic to infrared energy is only 50%. Half of the photons are trapped by the plastic, warming it up. The heated plastic emits infrared photons, some of which escape to the outside. But others are emitted back down inside the box where they will warm the floor still further.
The net result is that more of the sun’s energy is absorbed by the greenhouse than in the box with no windows. Air molecules inside the greenhouse thus have many more opportunities to touch the warm walls, floor, window, and top of the box, where they will be bumped and shoved so that they move even faster. Thanks to the window, the air inside the greenhouse warms up, and keeps the exotic plants from “catching cold.”
III. How the Earth’s Atmosphere “Captures” Solar Energy
The idea that the Earth is like a florist’s greenhouse is not quite accurate. You can always open the window of a greenhouse to let outside air flow in and the greenhouse will cool off. You can’t do that with the Earth’s atmosphere. The diagram on this page shows how the Sun’s energy flows through the Earth-atmosphere system.
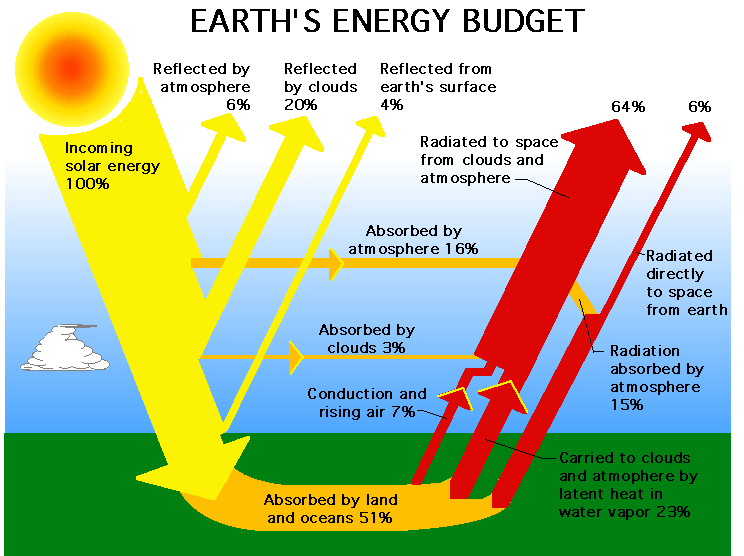
For the Earth to retain its energy balance, the amount of radiant energy absorbed must be equal to the energy radiated back into space. If this were not so, Earth’s temperature would rise continuously and the oceans would boil away. Our planet absorbs about 70% of the solar energy that it intercepts, and radiates the same amount to space. But the energy takes a slight detour along the way (refer to Earth’s Energy Budget diagram):
- Input—The Earth intercepts one billionth of the Sun’s energy output. This fraction of the Sun’s light energy powers the Earth-atmosphere system.
- Reflected—Measurements show that 25% of the energy intercepted by the Earth is reflected from cloud tops and particles of dust in the Earth’s atmosphere. Five percent is reflected from the Earth’s oceans, land, and polar caps. Thus 30% of the solar energy intercepted by the Earth does not enter the Earth-atmosphere system at all. Observers from other planets that might take a picture of us would see us by this reflected light.
- Absorbed—Twenty-five percent of the energy intercepted by the Earth is absorbed by the atmosphere, warming it up. Forty five percent is absorbed when it strikes the surface of the Earth, warming it further. Thus 70% of the energy intercepted by the Earth is absorbed by the Earth-atmosphere system.
- Greenhouse Effect—The heated oceans and land surfaces emit infrared photons. The atmosphere absorbs these photons and warms up. The atmosphere emits other infrared photons, some of which go back down to the Earth’s surface, warming it even further. This exchange of energy between the atmosphere and the ground is known as the greenhouse effect.
- Output—According to careful measurements, a small amount (4%) of the infrared energy radiated by the ground passes through the atmosphere without getting absorbed, and goes directly into space. Some of the infrared energy radiated by the atmosphere also escapes into space (66%).
The Energy Balance. All of the energy intercepted by the Earth returns to space. Some of it is reflected in the form of visible light (5% + 25% = 30%), while the rest is emitted in the form of infrared energy (4% + 66% = 70%). In the meantime, temporarily trapped energy keeps the Earth’s surface at a nice living temperature.
The Greenhouse Effect
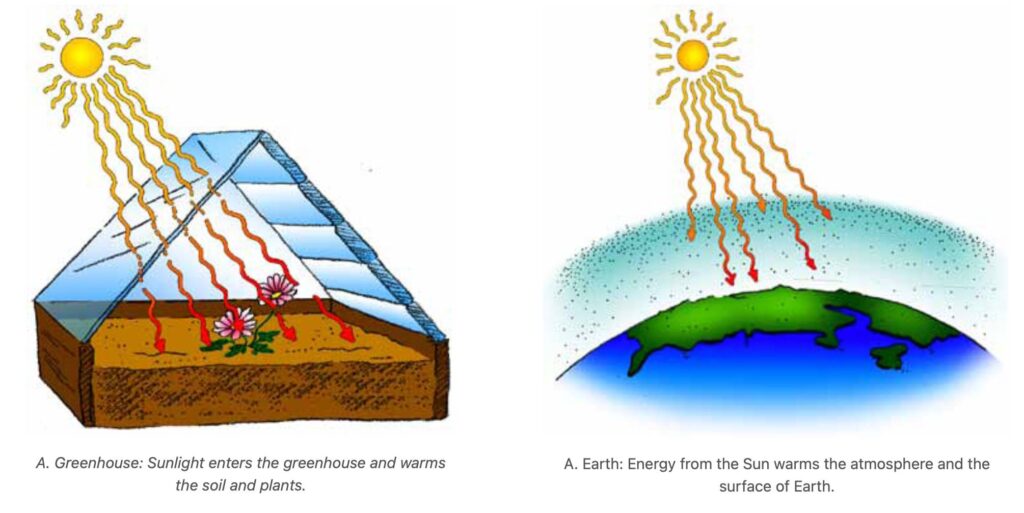
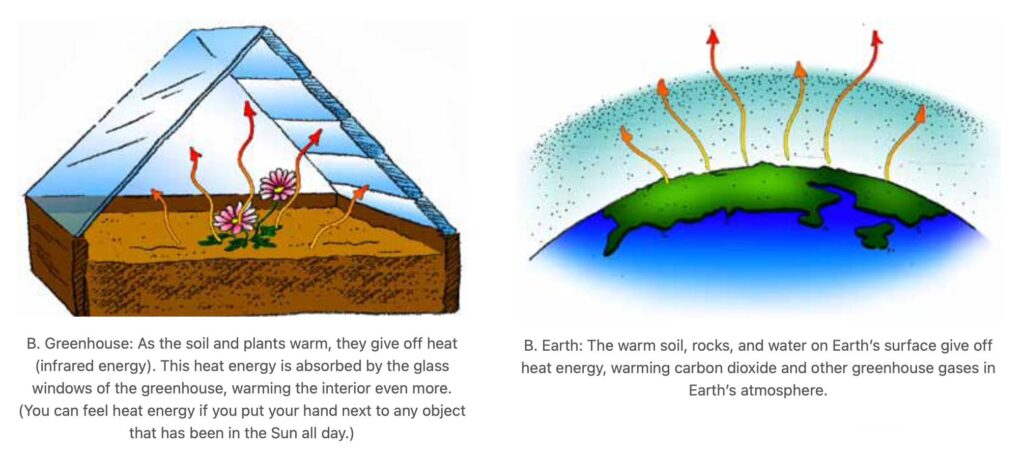
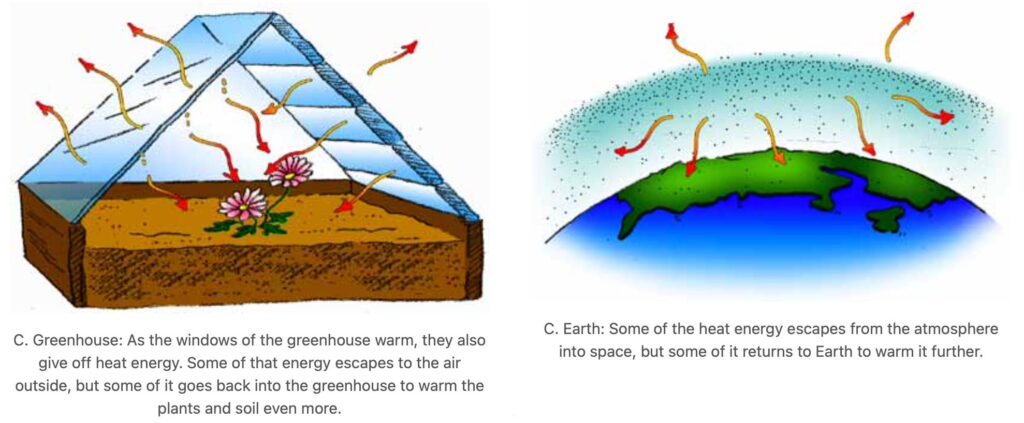
.
Now please recall the Equilibrium Investigation you did (EF6.1).
IV. Greenhouse Gases and the Goldilocks Effect
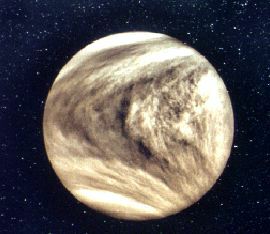
Venus is too hot!
Because it has a dense atmosphere of carbon dioxide and no oceans, Venus has a greenhouse effect that raises its temperature by 483°C. (NASA photo)
Mars is too cold!
Because Mars is much smaller than Earth and Venus, it cannot hold onto a dense atmosphere, so the greenhouse effect on Mars is only 6°C. (NASA photo)
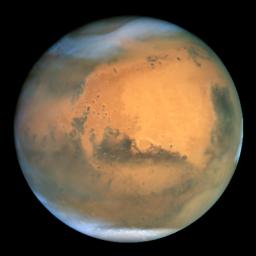
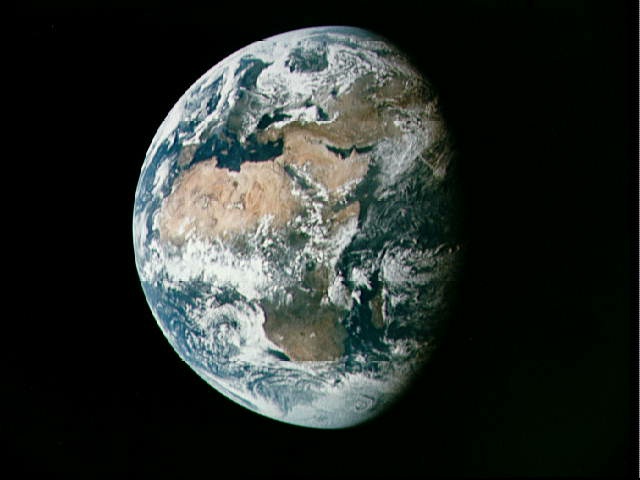
Earth is just right!
Thanks to our oceans and an atmosphere with about 0.03% and small amounts of other greenhouse gases, the Earth’s greenhouse effect is about 33°C. (NASA photo)
If you travel in a rocket ship to the upper reaches of the Earth’s atmosphere, you’ll find that it’s very cold up there: about -18°C below zero. But here at the surface, it’s a comfortable 15°C above zero. What accounts for the difference?
Some gases in the Earth’s atmosphere are more efficient at absorbing infrared radiation than other gases. The very efficient infrared absorbers are called greenhouse gases, and include mainly carbon dioxide, water vapor, methane, and a few others. The warming effect, 33°C, is due to the presence of greenhouse gases in the atmosphere. If it were not for these greenhouse gases, the Earth would not be warmer at the surface, and we’d be locked in a perpetual ice age.
Mars is further from the Sun than the Earth, so we’d expect it to be colder. The top of the Martian atmosphere is -66°C below zero. If we land, we’d find that the greenhouse effect on Mars is very slight, warming the planet just 6°C; so the average temperature on the surface of Mars is -60°C—not the best place for a vacation. The reason for this is that Mars lost most of its atmosphere billions of years ago. Its current atmospheric pressure is less than a hundredth that of Earth and its greenhouse effect is just barely measurable.
Venus is closer to the Sun than the Earth, but it has bright clouds that blanket its surface and reflect 80% of the Sun’s energy so it absorbs less solar energy than the Earth. The cloud tops are a cool -23°C, which is a little cooler than the Earth. However, its dense atmosphere of carbon dioxide is so efficient at trapping heat that the temperature at the surface has been measured at 460° C! That’s a greenhouse warming effect of 483°C! This has sometimes been referred to as a “runaway greenhouse effect” that can occur if a planet’s greenhouse gases are abundant enough. It is so hot on the surface of Venus that lava from volcanoes flows for hundreds of miles before it solidifies.
Venus, Mars, and Earth are all at equilibrium. They emit just as much energy into space (in the form of infrared energy) as they absorb from the Sun. However, each of these planets has a very different greenhouse effect because their atmospheres are very different. If we were really visitors from space, we’d find that Venus is much too hot for habitation; while Mars is much too cold. Earth is the only planet that is just right! This has come to be called “The Goldilocks Effect,” after the fairy story, Goldilocks and the Three Bears. If we want Earth to continue to be “just right,” we need to be certain that the level of greenhouse gases does not increase too much beyond the current level.
Planet Name | Measured from Space | Greenhouse Effect | Measured at Surface | Goldilocks says, It’s |
---|---|---|---|---|
Venus | -23 °C | +483 °C | 460 °C | too hot! |
Earth | -18 °C | +35 °C | 15 °C | just right! |
Mars | -66 °C | +6 °C | -60 °C | too cold! |

V. The Global Warming Hypothesis
The graph on this page represents the Earth’s average global temperature since weather station measurements began to be recorded systematically in 1850. Each point on the graph represents an average made at hundreds of stations all over the Earth, every day for a year.
The data show that the Earth has been warming up in the past century. The question arises, what caused the warming? Many scientists believe that increasing concentrations of greenhouse gases, especially CO2, associated with burning of fossil fuels since the time of the industrial revolution, is leading to global warming. Earth could experience a 1°C to 3.5°C increase in temperature in the next 100 years. Although this does not sound like much, it could cause climate changes having serious consequences for many creatures on Earth, including humans.
VI. Opponents of the Global Warming Hypothesis
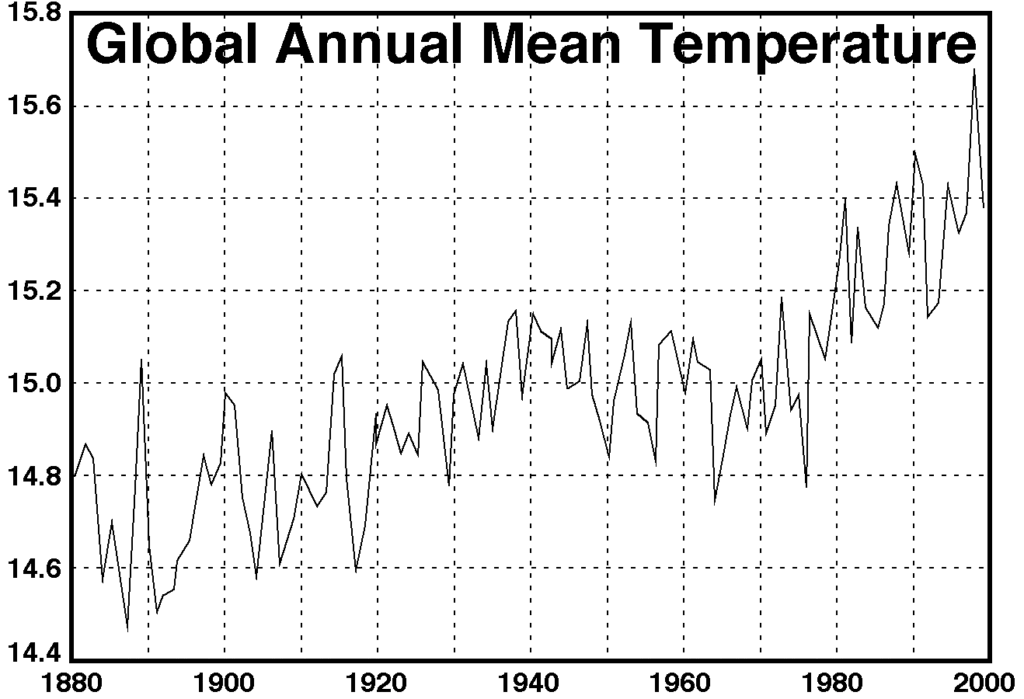
Close examination of the data show that the Earth was warming up in the 1890s and continued to do so until the 1940s when there was a cooling. It wasn’t until the 1980s that the warming trend started again. Although clouds of particles from volcanic action are cited as the reason for the cooling period of the 1940s, the cooling has not yet been fully explained.
Scientists are skeptics. That is, they generally resist accepting things that they are told just because someone, even someone who is said to be an “authority,” says it is true. A scientist wants evidence: measurements or observations, which have been carefully collected so that they can be analyzed and independently evaluated before a conclusion can be agreed to.
Since 1988 scientists have hotly debated whether or not the gradual warming of the Earth’s climate, by about 1°C over the past century, is due to the release of greenhouse gases by human activities. In December, 1995, the International Panel on Climate Change (IPCC), a United Nations team of 2,500 scientists from around the world, cautiously concluded that “The balance of evidence, from changes in global mean surface air temperature and from changes in geographical, seasonal and vertical patterns of atmospheric temperature, suggests a discernible human influence on global climate.”
VII. Unknowns
Although the vast majority of climatologists are convinced that an increase in greenhouse gases will eventually result in an increase in the Earth’s average annual temperature, there is a lack of agreement on when or how fast that global climate change may happen. Predictions are based on computer models which leave out many details of global climate factors and their predictions vary so much that long range estimates are not reliable. Much is unknown about how Earth systems process the energy emitted from the Sun.
Even if we assume that warming will occur as greenhouse gases increase, keep in mind that the changes will not occur today, probably not tomorrow, or possibly not even within our lifetimes. The predictions are that the climate will warm over the next century. In other words, our current uses of fossil fuels is likely to affect the climate for our children and our grandchildren.
While virtually all climatologists agree that the theory of global warming caused by an increased greenhouse effect is correct, there is a great deal of disagreement with regard to specific questions. These questions define the frontier of research on global climate change. For example:
- Does the amount of energy emitted by the Sun change over time?
- To what extent will the destruction of rain forests increase the amount of carbon dioxide in the atmosphere?
- How will these factors change the climate?
The largest unexplored frontier on Earth is the ocean which covers three quarters of its surface. Water can absorb large quantities of heat and carbon dioxide gas. Ocean currents distribute the heated surface water of the tropics around the globe and into the depths.
There is much to be learned about how the ocean will respond to global warming. One scenario suggests that a warmer ocean may not absorb as much carbon dioxide as it presently does. This would be a feedback effect which would add to the severity of global warming and is therefore called a positive feedback effect.
On the other hand, a warmer ocean and atmosphere means more evaporation of surface water and an increase in the production of clouds. Will the additional clouds shade the surface and reflect more of the Sun’s energy back into space? This would be another feedback effect but one which would work in the direction of reducing the warming. It is a negative feedback. It is known that low flying clouds do cool the surface but high thin clouds tend to increase the greenhouse effect. There is simply not enough known about the role of clouds to definitely say what their effect will be.
The greatest uncertainties, however, concern how much the climate will change in different regions, and what effect this change will have. Predictions of very long hot dry spells, droughts in some areas, floods in other areas, and more intense storms in still others, remain uncertain. On these issues, the jury is still out. The systems are so complex, global warming will make an interesting and vital issue to watch in years to come.
VIII. Conclusion: Is Earth’s Equilibrium Changing?
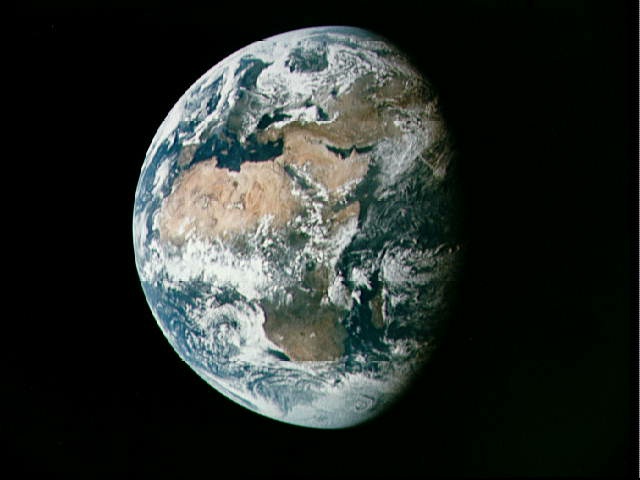
In the water bottle demonstration, as long as the water level in the bottle is constant or varies within limits, the bottle is in equilibrium. On average, the water flowing in must equal the water flowing out. In a similar way, the Earth will continue to emit into space as much energy as it receives from the Sun. To a space visitor who measures only the total amount of infrared energy emitted from the Earth, the greenhouse effect will not be noticed. However, from our vantage point, the prediction of global warming is that the average temperature at the Earth’s surface will increase. What we don’t know is how much it will warm, when the warming will occur, and what effect it will have.
Following our bottle analogy, the climatologists’ question is like trying to predict what will happen if one of the holes at the bottom of the bottle is blocked. It is not easy to accurately predict unless we try the experiment. And that is just what the human species is doing—conducting an experiment with the entire atmosphere of our planet as a test chamber.
In a sense, the puzzle of global warming and the greenhouse effect is like the question about why the Eastern and Western halves of the Pacific Ocean floor look so different (Chapter 3, p. 32). Both are fascinating questions about changing conditions on our planet. Both involve observation and measurement of the available evidence and both involve knowledge about how energy flows through natural systems. Furthermore, knowledge about how our planet’s climate changed in the past provides valuable information about how the climate may change in the future. Although the sources of energy might be different (burning of fossil fuels rather than volcanic eruptions) the ways that energy flows in the two situations may be the same.
In the next chapter, we’ll turn to the short-term changes caused by the flow of solar energy in our atmosphere—the complex variety of energy flows that we call “weather.”