OZ5. A Mystery Solved
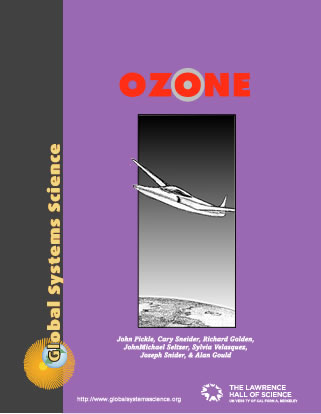
Chapter 5
{ Ozone Contents }
I. The Scientific Investigation
Jim Lovelock is an unusual person in today’s science community. He does not work at a university nor is he part of any business organization. He works out of a small laboratory in his home in the quiet countryside of Wilshire, England, and he works alone. Independent scientists were common in the 1800s, but they are quite rare today.
During the late 1950s Dr. Lovelock designed a very sensitive instrument that made him famous. The instrument is called an electron capture detector, a device that allows tiny amounts of different gases in the atmosphere to be detected. The instrument is so sensitive it can detect the presence of a gas even when there are only a few molecules of it in a trillion molecules of air. It can be used to detect traces of chemical pollution in solid substances, and discoveries made with the instrument showed that in every animal on Earth there are traces of man-made chemical pollutants. The evidence provided by Lovelock’s invention enabled Rachel Carson to justify her concern about the dangers of DDT in her famous book Silent Spring in 1962.

Lovelock’s Electron Capture Detector in Gas Chromatography
Chromatography is the separation of a mixture of compounds into individual components for identification. Gas chromatography is one technique used to identify compounds that are vaporized and passed through tiny tubes, called capillaries, filled with different materials that impede the movement of individual gases, allowing separation of the gases. The compounds must be stable when heated to form a vapor; otherwise new materials would be identified. Lovelock’s instrument made detection of the vapors created in gas chromatography more accurate.
In 1970 Lovelock turned his instrument to the study of chlorofluorocarbons. To the surprise of everyone he detected significant amounts of CFCs in the air over western Ireland as the air came in from the west. It was a surprise because most pollutants are broken down rapidly in the troposphere or are removed by rain, but here were molecules that had traveled across the Atlantic Ocean. Lovelock decided that CFCs would be perfect for tracing the flow of air masses in the atmosphere. Since only the industrialized countries released CFCs, his instrument could be used to identify the origin of a particular air mass. During 1971 and 1972 he took his instrument on board a research vessel traveling between Britain and Antarctica. He found that the concentration of CFC-11 and CFC-12 ranged from a few to 230 parts per trillion. That’s roughly the same as if a drop of liquid CFC were diluted in the volume of a large swimming pool.
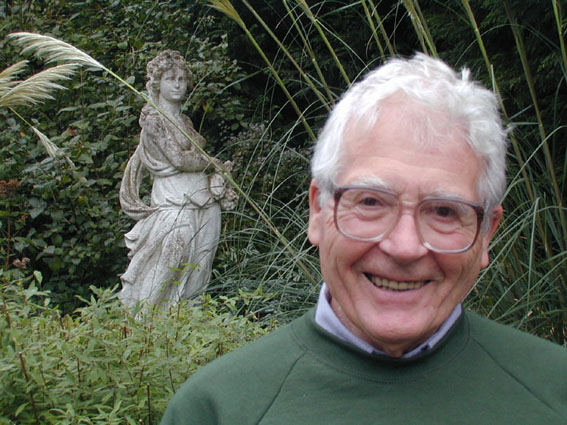
Lovelock, Gaia, and Mars
When NASA was designing its unmanned Viking mission that would go to Mars to search for life, it called upon Lovelock to help design its instruments. Lovelock turned out to be a severe critic of the program. He complained that the proposed series of Martian experiments were based on a false assumption. He said scientists were preparing to look for evidence of life on Mars in the same way that they would examine the soil on Earth for evidence of life. The Martian explorer was to scoop up samples of the soil and analyze it in an automatic laboratory. Lovelock asked, “How can we be sure that the Martian way of life, if any, will reveal itself to tests based on Earth’s life style?” He predicted that the instruments of the Martian explorer would not find any sign of life. It didn’t. But we still do not know if Martian life would reveal itself with other detection techniques, as Lovelock implies.
In his book, Gaia: A New Look at Life on Earth, Lovelock explains that his NASA experience got him thinking about a more difficult question, “What is life, and how should it be recognized?” He expected to discover in the scientific literature some definition of life so that he could design a life-detection experiment. He was disappointed. He was surprised how little was written about the nature of life itself. Thinking about life on Mars gave Lovelock a fresh view of how one might look for life on Earth. That view resulted in an interesting and controversial hypothesis.
To Lovelock it seemed that all living systems on Earth were part of one gigantic system which itself was alive. This system he called Gaia, after the Greek goddess of life. The Gaia hypothesis states that conditions for life on Earth are maintained by the living systems themselves. These living systems operate automatically and unconsciously by way of active feedback processes.
Whether or not the Gaia hypothesis is correct is being argued by scientists around the world. Whatever the fate of the idea, it has already proved valuable because it has helped to emphasize the interdependence of all of Earth’s living systems.
Lovelock wrote up the results of his investigation and in 1973 it was published in the British scientific journal Nature. In the paper he made a statement that he would later find to be completely wrong, saying, “the presence of these compounds constitutes no conceivable hazard.” They seemed unchanging and unchangeable and therefore no danger to anyone.
II. Sherwood Rowland and Mario Molina
The leading producer of CFCs in the world is the Du Pont Company. In 1971 a meeting between Jim Lovelock and Ray McCarthy, the chemist who ran Du Pont’s CFC laboratory, resulted in one of two chance conversations that changed the whole course of atmospheric research. Based upon his studies, Lovelock had estimated the tonnage of CFCs that were floating in the air around the world. He asked McCarthy how many tons of CFCs he would estimate had evaporated since CFCs had been manufactured. To both men’s surprise the figures were just about the same. The implication was that nothing had happened to the CFCs in the air. Very little, if any, had been destroyed.
A few months later Sherwood Rowland, a chemist specializing in radioactivity at the University of California at Irvine, was strolling among the scientists during a coffee break at a scientific meeting. He chanced to hear the story of the meeting between Lovelock and McCarthy. It stuck in his memory because it was his belief, based on his knowledge of the action of sunlight, that the CFCs should be broken up in the atmosphere.
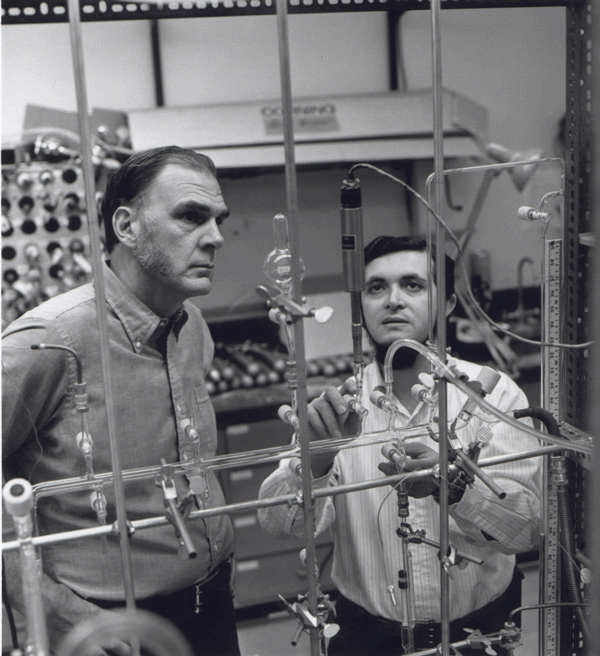
The next character in our story is young Mario Molina who had just completed his Ph.D. studies in laser chemistry at the University of California at Berkeley. He wanted to continue research work under Sherwood Rowland and so he went to Irvine to speak with him.
Sherwood Rowland and Mario Molina in their laboratory at the University of California Irvine during the 1970s.
(Photo provided by Dr. Sherwood Rowland.)
During their discussion Molina found out that Rowland felt that he had exhausted himself in the study of radioactive chemicals and was planning to take some time off to consider what he might study next. Disappointed, Molina asked Rowland if there were any interesting problems that he might investigate. Rowland remembered the conversation he had overheard about the persistence of CFCs in the atmosphere and suggested that Molina might try to find out what was happening to the CFCs. Rowland said he thought ultraviolet energy from the Sun would break the CFCs apart, and was therefore surprised that the amount of CFCs seemed to remain constant. Mario Molina was intrigued with the problem and set to work.
At that time a significant source of CFCs being released into the air occurred when something went wrong with a refrigerator or air conditioner. The first thing a repairman would do was to open a valve to allow the liquid CFCs inside to evaporate into the air so the pressure inside the system would be reduced. Another source of CFCs was spray cans. Most of them were powered by inserting CFCs with the product to be sprayed. When the product was exhausted the gas escaped as the user pressed the button on the can. The can was then discarded and when it broke or was crushed the remaining CFCs were released into the air.
Molina found, at least in the lower atmosphere, that nothing happened to the molecules of CFCs. They would continue to move about quite randomly, undergoing occasional collisions with other molecules, until they became distributed throughout the troposphere. This explained why the amount of CFCs released and the amount found in the atmosphere was about the same. However, the CFC molecules did not remain in the lower atmosphere forever. Because of their long lifetimes the CFC molecules could eventually be transported up into the stratosphere.
By careful experimentation Molina showed that Sherwood Rowland’s hypothesis was correct. In the stratosphere, before the highly energetic ultraviolet energy from the Sun could be absorbed by ozone, it was breaking up molecules of CFCs. Rowland and Molina examined the chemistry involved. The net result of the breakup of CFCs was the release of chlorine atoms. They found the chlorine atoms were very effective in breaking up molecules of ozone. All the elements of a global problem were there. CFCs could get into the stratosphere where there was plenty of ultraviolet radiation and where the Earth’s protective ozone shield resided. Could the CFCs seriously affect the ozone layer?
Preliminary calculations by Rowland and Molina showed that the amount of CFCs that could have reached the stratosphere was exceedingly small and the amount of ozone that it could destroy was so slight as to be negligible. They had answered their original question. “What happens to the CFCs in the atmosphere?” The answer was, “Most of the time—nothing. But those CFC molecules that got into the stratosphere were broken up by the Sun’s ultraviolet radiation and chlorine atoms were released.” They found that those chlorine atoms would break up ozone and, as so often happens in science, answers provoked further questions. They continued their investigation.
III. Where Did the Pieces Go?
“What happened to the broken-up ozone? Where did the chlorine go?” Molina and Rowland knew how important ozone was in protecting life on the Earth’s surface and their concern about any loss of ozone prompted them to continue their work.
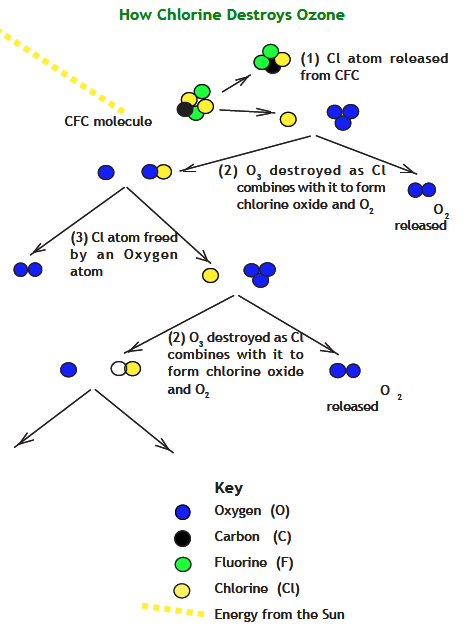
Based on their investigations Rowland and Molina proposed the following scenario for the upper atmosphere chemistry of ozone and CFCs: (In this example CFC-12 (CCl2F2) is used.)
CCl2F2 + hnUV ⇒ Cl + CClF2
In plain English, this chemical equation says CFC-12 plus ultraviolet energy produces a free chlorine atom (Cl) and a chlorine compound (CClF2). Now a molecule of ozone comes along.
Cl + O3 ⇒ ClO + O2
The free chlorine atom breaks up the molecule of ozone by combining with it to form chlorine monoxide and oxygen, and one molecule of ozone is destroyed.
ClO + O ⇒ Cl + O2
Chlorine monoxide can combine with single atoms of oxygen, which are generated by the action of UV energy on dioxygen and ozone molecules, to produce a free atom of chlorine and a molecule of oxygen. This process is diagrammed on this page. Study the reactions until you can extend the diagram. Then read on!
Question 5.1. What happens next?
Using the diagram on this page, place the top edge of a sheet of paper on the dashed line and continue the diagram showing how a single chlorine atom can destroy more and more ozone molecules. How many times can the reaction be drawn before the process stops?
When the chlorine release step was worked out, Rowland and Molina realized that ozone in the stratosphere was in trouble. Their first estimate of negligible loss of ozone was incorrect. As the reactions continued, the chlorine atoms emerged unaffected and ready to destroy other ozone molecules. They could do this time after time. Molina and Rowland calculated that a single chlorine atom released from a CFC molecule could go on to destroy as many as 100,000 ozone molecules before the atom was transported out of the stratosphere. The ozone layer was under attack.
IV. Adding the Effects of CFCs on Ozone Molecules
Review the ozone/oxygen “dance” on page 17. The natural system kept the ozone level fairly constant by a negative feedback process. The addition of CFCs to the system has increased the number of dancers. In this dance, chlorine is the new partner that keeps cutting in, breaking up ozone trios and releasing oxygen molecules. Just a few CFC molecules can provide the chlorine atoms that can destroy hundreds of thousands of ozone molecules.
In chemistry when something acts to speed up a chemical reaction without itself being changed in the process it is called a catalyst. In this case the chlorine atom is the catalyst of ozone destruction.
V. Not All CFCs are Created Equal
Not all CFCs have the same number of chlorine atoms, so if the CFC releases all of its chlorine into the stratosphere, there will be different amounts of chlorine released. The strength of chemical bonds also differs with each species of CFC, so the amount of chlorine each can release varies. One way to quantify the amount of ozone each CFC species can destroy in the stratosphere is to relate it to that of an equivalent mass of CFC-11. If one kilogram of the CFC species destroys the same amount of ozone as one kilogram CFC-11, then the Ozone Depletion Potential (ODP) is 1. If it can destroy twice as many ozone molecules as an equal mass of CFC-11, then the ODP = 2. If it can destroy half as many ozone molecules, then the ODP = 0.5.
Examples of ozone depletion potential for several chemical species of CFCs are in Table 4.1. Lifetime represents length of time it takes for 63% of the original amount of the chemical to be removed from the atmosphere. Therefore, if the species has a 45-year lifetime, on average, 37% of the original amount of the chemical is remaining in the atmosphere after 45 years. To compare, the lifetime of ozone in the upper troposphere is roughly several weeks, whereas, near ground level ozone’s lifetime is on the order of minutes.
Table 4.1 Ozone Depletion Potentials
Chemical name & Formula | Lifeline in the atmosphere (years) | Ozone Depletion Potential (ODP) |
---|---|---|
CFC-11 CCl3F | 45 | 1.0 |
CFC-12 CCl2F2 | 100 | 1.0 |
CFC-13 CClF3 | 640 | 1.0 |
CFC-113 C2F3Cl3 | 85 | 0.8 |
CFC-114 C2F4Cl2 | 300 | 1.0 |
CFC-115 C2F5Cl | 1700 | 0.6 |
VI. Additional Ozone Destroying Substances
There are chemicals other than CFCs that can destroy stratospheric ozone. These chemicals are called Ozone Depleting Substances (ODSs) and include bromine compounds, such as halons (used in fire extinguishers), methyl bromide (used as a fumigant for global distribution of farm produce), and carbon tetrachloride (wide number of uses, but after the mid-1960s, used primarily as an insecticide). Bromine compounds have particularly high ODPs since bromine is about 50 times more efficient at destroying ozone than chlorine released from CFCs. The effects of these chemicals were identified after the discovery of the link between CFCs and stratospheric ozone, but they are also important to control for the protection of the stratospheric ozone.
Examples of ozone depletion potential for several chemical species of non-CFCs are in Table 4.2. Notice that the several species, in particular bromine-containing materials, have a remarkably high ODP.
Table 4.2 Ozone Depletion Potentials for several types of Ozone Depleting Substances
Chemical name & Formula | Lifeline in the atmosphere (years) | Ozone Depletion Potential (ODP) |
---|---|---|
Halon 1211 CF2ClBr | 11 | 3.0 |
Halon 1301 CF3Br | 65 | 10.0 |
Halon 2402 C2F4Br2 | – | 6.0 |
Carbon Tetrachloride CCl4 | 35 | 1.1 |
Methyl Chloroform C2H3Cl3 | 4.8 | 0.1 |
Methyl Bromide CH3Br | 0.7 | 0.6 |
VII. The Spray-Can War — The Public Won, Didn’t It?
After Rowland and Molina published their theory of ozone destruction, they presented it at the 1974 meeting of the American Chemical Society. Their presentation received coverage in newspapers and information about their ideas spread rapidly. Chemical manufacturers and users of CFCs were quick to respond. They pointed out that there was no evidence of chlorine in the upper atmosphere and that, although no adverse consequences of CFC use were known, more research would be a good idea. Nineteen companies formed the Chemical Manufacturers Association’s Fluorocarbon Program Panel. To their credit this group helped finance scientific research into the fate of CFCs in the atmosphere, including partial funding for the Antarctic and Arctic expeditions which were to follow in 1986 and 1987.
Despite Rowland and Molina’s disturbing warning in 1975, the global production of CFCs throughout the early 1980s continued at almost the same level, and new uses were being found. Scientists were more cautious than the manufacturers, and their concern about the growing use of CFCs as propellants in spray cans led many of them to call for a ban on such use. Basically, they were worried that increased ultraviolet light reaching the surface of the Earth would lead to increased risk of skin cancer, some forms of which are quite deadly.
But American consumers began to boycott spray-can products using CFCs—air sprays, paints, deodorants, insect killing chemicals, whip cream—there were hundreds of products packaged in cans with the little button on top. Sales of CFC-containing aerosol containers began to fall off.
In 1975 several scientists testified before Congressional committees that damage to the ozone layer seemed probable. Their testimony produced several bills to ban the use of CFCs in aerosol containers, but they were defeated. The scientific evidence was not strong enough. The theory that CFCs would release chlorine, which would then affect the ozone layer, was not supported by hard evidence. Committees were established to evaluate the role of CFCs in ozone destruction.
In September 1976 a report from the National Academy of Sciences called for prompt action to be taken by the United States. The report predicted that even if CFC emissions were held to 1973 levels it would still lead to a 6% to 7.5% reduction in the amount of stratospheric ozone. This in turn would mean a 12% to 15% increase in the amount of dangerous ultraviolet radiation reaching the Earth, increasing the danger of skin cancer, cataracts, and other problems.
In March 1977, Oregon banned aerosol cans containing CFCs. New York followed by requiring warning labels to be put on aerosol cans that had CFCs, declaring the product to be a hazard to ozone. In May, 1977, the Environmental Protection Agency (EPA) established regulations that, in effect, banned the use of CFCs in aerosol cans. The regulations took effect in March 1978. Only four years had passed since Rowland and Molina first gave their warning.
Today all aerosol spray cans made in the U.S. use propellants that are harmless to the ozone layer. Since the United States was responsible for almost half of the total global use of CFCs these actions produced a worldwide decline in CFCs for a few years. But new products using CFCs made their appearance during the 1980s: cleaning electronic circuit boards by dipping them into liquid CFCs; most every new car had air conditioning units. Demand for CFCs continued to rise even though the use of CFCs as propellants declined.