EF4. How Does the Sun Shine?
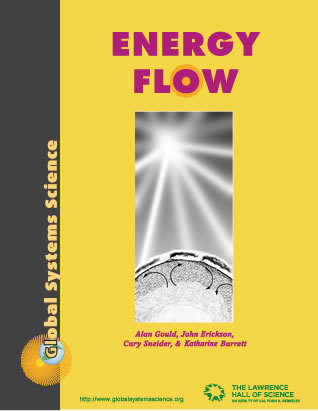
Chapter 4
An outdoor expedition once brought a group of high school students to the top of a bare plateau in the middle of the night. Even though it was July, the night air was cold and the wind was blowing strongly. The wind thwarted their efforts to build a fire. They couldn’t sleep, and for hours they huddled together to keep warm. As the sky finally lightened and the stars winked out, they watched as the Earth turned and slowly brought the Sun into view. When the first rays of light shone over the distant horizon, they burst into spontaneous applause and “hoots” of appreciation. Soon the rays of sunlight, now exploding into brilliant colors all over the sky, would bring the warmth that they and every other living thing depended upon for survival.
I. The Sun—An Average Star
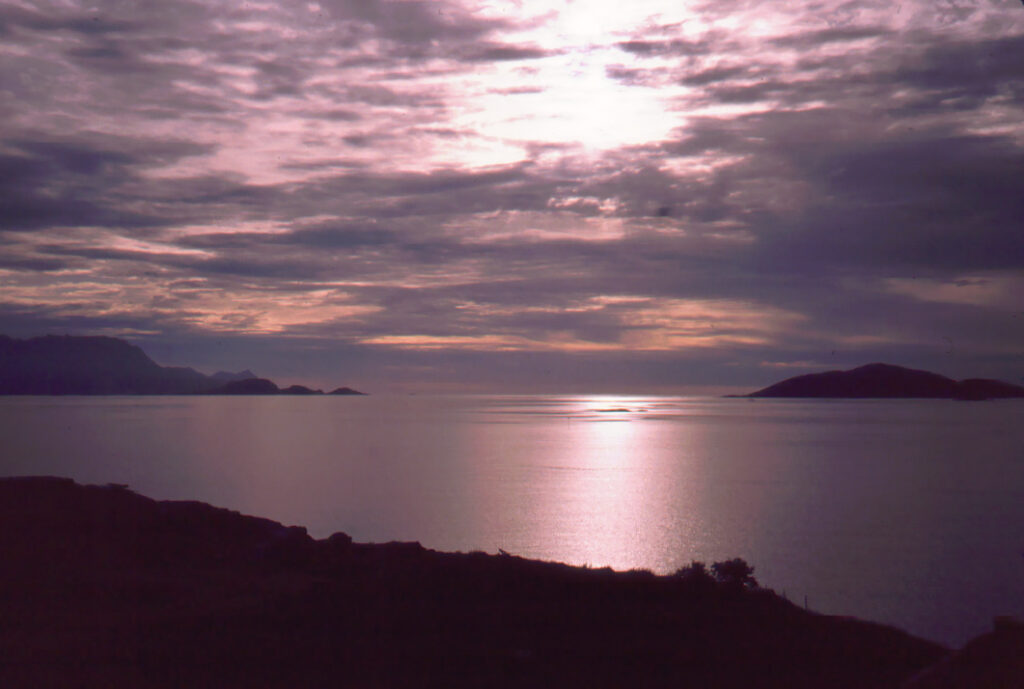
Photo by Alan Gould, Lawrence Hall of Science
Until now we have focused entirely on the sources of energy within the Earth—the heat energy generated by gravity when the Earth first formed, and the energy produced by nuclear fission from radioactive materials deep underground. In this chapter we’ll focus on the third big source of energy pouring into the Earth system—the Sun.
The Sun is a star. It’s about 100 times the diameter of the Earth.
If the Sun were hollowed out, one million Earths would fit inside it. As stars go, it’s not unusually big or unusually small; nor is it unusually bright or dim. It’s an average star—one of between 200 and 400 billion stars in a huge pinwheel of stars called the Milky Way galaxy—which is in turn one of countless numbers of such galaxies in the Universe.
Although the Sun is not an unusual star, its power is awesome. The Earth intercepts only a tiny amount of the total energy that the Sun produces. Yet that energy powers all movements of wind and waves; it lifts up to the sky all the water that falls back to the ground as rain or snow; it warms all jungles and the deserts; it provides the energy for all plants to manufacture their food, which in turn supports all animal life, including us; and… it warms our faces and hands after a cold night outdoors. All this energy captured from the Sun in Earth systems is only about one billionth of the total energy output of the Sun! Yes, the Sun’s power is awesome.
II. A Visit To Our Star
Temperatures on the Sun range from 6,000°C at the visible surface, to over 15 million °C at the core. Given these conditions, you should probably do as Earth-based astronomers do—study the Sun from a safe distance and infer what it is like inside by computer modeling. But if you could visit the Sun, you might see something like the pictures on the next two pages.
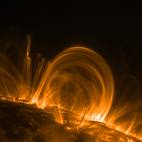
Courtesy of NASA
Imagine you are visiting the Sun, equipped with a comfortable heat-protective suit, exceptionally strong sunglasses, and a powerful rocket engine to get you home again. Poised above the Sun’s visible surface in a small spacecraft, you see the faint, wispy streamers of the corona all about you. This is the outer atmosphere of the Sun, which extends far beyond Earth, and even beyond Pluto. Streamers of charged particles—atoms that have been torn apart into electrons and protons—follow the magnetic field lines of the Sun, in patterns like iron filings on a sheet of paper covering a bar magnet.
Arching above the Sun’s surface are very bright streamers of hot gas called prominences. Material in these huge loops also follow the magnetic field of the Sun, most of it falling back to the surface, but some escaping to become part of the corona.
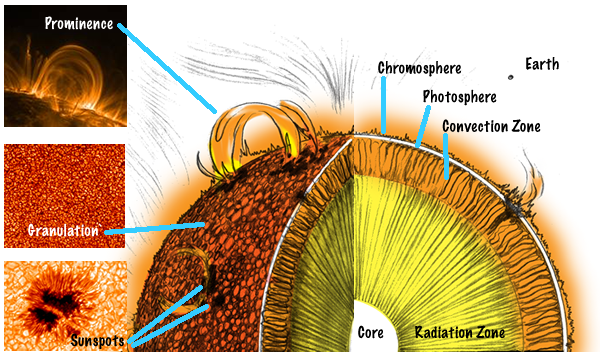
Rising everywhere from the Sun’s visible surface are finger-like streamers of hot glowing gas called spicules. These comprise the lower atmosphere of the Sun, called the chromosphere. The name comes from the Greek word “chromos,” which means “color,” and refers to the beautiful pinkish hues seen during a Solar eclipse, when the bright parts of the Sun are obscured so we can see its atmosphere.
Before descending to the surface, take a sample of the solar atmosphere. You’ll find that the charged particles are moving very, very fast. Recalling that the kinetic energy of individual particles is measured as “heat,” you’ll probably conclude that the solar atmosphere is very hot—millions of °C! However, the particles are few and far between. In fact, there is so little “stuff” in the solar atmosphere, that it would be considered a nearly-perfect vacuum on Earth! If there were a breach in your spacecraft hull, you would not be burned by the very thin atmosphere—you would die from suffocation, decompression and radiation.
Now donning your solar space suit, you leave your comfortable spacecraft and descend to the Sun’s visible surface—the photosphere. As you descend towards the surface, you notice that it is completely covered with bright regions, about the size of Wyoming, surrounded by darker areas. This is called “granulation.” Each granule is the top of a convection current. Hot gas comes up in the bright center portion, releases its energy and cools, then sinks along the edges of the granule.
Now coming in for a “landing” on the photosphere, you immediately sink! That’s because there is no solid surface. The atoms are still so far apart here that the pressure is roughly one sixth of the pressure of air at the surface of the Earth. Checking your thermometer, you find that the temperature in this region is “only” about 6,000°C.
By the time you reach the bottom of the convection zone, you will have fallen about 15% of the way towards the Sun’s center, and the temperature will have climbed to about 500,000 °C. As you fall, the density increases. At the bottom of the convection zone, density is about 1% of water on Earth.
You have now entered the radiation zone. At this depth there are no more atoms—only pieces of atoms—mostly electrons and protons floating in a “particle soup” called plasma. The material around you becomes more transparent, and you can see that it is brighter towards the center of the Sun. You continue to sink into the radiation zone.
Your descent finally halts about half-way to the center of the Sun, when the density of the Solar plasma reaches the density of your body, which is composed mostly of water. Assuming your suit is still protecting you, you will find that the temperature is now millions of degrees Celsius. [See explanation of temperature scales.] If you could dive deeper, you would enter the core, where it is hot enough for hydrogen to fuse. At the Sun’s center, you’d find the temperature to be about 15 million °C and the density to be about 12 times that of lead. But if you’re smart, you’ll push the panic button, igniting the rockets strapped to your back to return you to your spacecraft, and read about what is going on in the core, rather than experience it first-hand.
III. The Secret of the Sun’s Furnaces
When the process of nuclear fission was discovered at the beginning of the twentieth century, some scientists proposed that perhaps the decay of heavy elements, such as uranium inside the Sun, produced its energy. This seemed reasonable, since heat inside the Earth is generated by radioactive decay as described in Chapter 3 (page 25). However, using instruments called spectrometers, that analyze the color of light from the Sun, astronomers found that it is composed mostly of hydrogen (70%) and helium (28%). Just 2% of the Sun is composed of heavier elements, and almost none of it is of the variety that will generate energy by radioactive decay.
So if radioactive decay is not what’s happening in the Sun, what is happening?
As luck would have it, there is a different kind of nuclear reaction, called nuclear fusion, where lighter atomic nuclei fuse together to form heavier nuclei. One type of nuclear fusion reaction is the combination of four hydrogen nuclei to form a single helium nucleus.
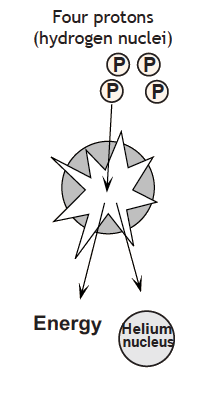
In reality, there is not one nuclear reaction, but a series of several different nuclear reactions.
See the GSS book A Changing Cosmos for more about secrets of the Sun’s furnace and how those secrets were discovered.
Careful measurements of mass show that the combined mass of four hydrogen nuclei is a tiny bit more—less than 1% more— than the mass of the helium nucleus after the nuclear fusion happens. The tiny amount mass does not just disappear—it is converted into energy. The amount of energy produced when mass is apparently “lost” can be calculated from Einstein’s famous equation:
E = mc2
In the most simple terms, this equation says that a certain amount of energy (E) is equivalent to a certain amount of mass (m) multiplied by the speed of light squared. The speed of light is a very big number: about 300,000 kilometers per second. If you could go that fast, you could go around the world seven times in a single second. So, indeed, the speed of light is a very large number, and when it is squared it is way bigger! So the tiny bit of mass that is lost when four hydrogen atoms fuse and become one helium nucleus results in a huge amount of energy.
And there is no shortage of hydrogen in the Sun. In the Sun’s core, about five billion kilograms of hydrogen is turned into helium every second and about 50 million kg of mass is lost each second—enough to account for the Sun’s production of energy. The Sun has been fusing hydrogen at this rate for about five billion years; and there is enough hydrogen fuel to keep the Sun “burning” for another five billion years.
IV. How Does Energy Get from the Sun’s Core to the Surface?
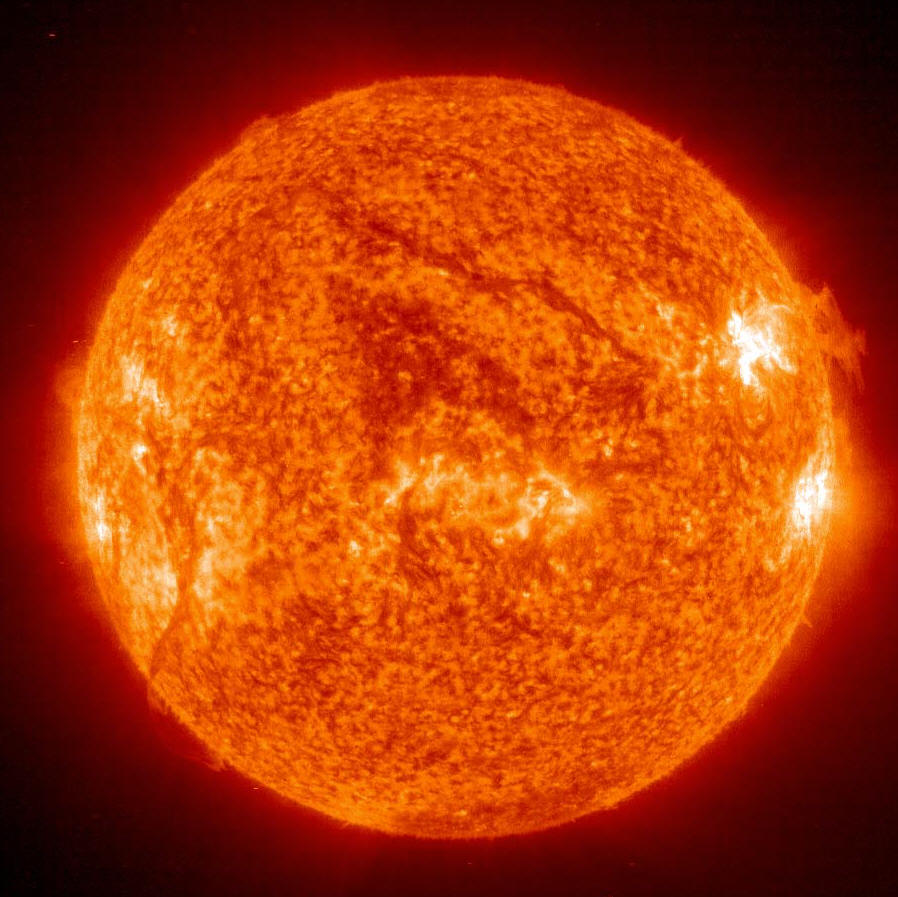
As shown in the diagram section II, the Sun’s core is relatively small, about 1% of the Sun’s volume. Within the core, the density is twelve times greater than that of lead, and the temperature reaches 15 million °C. These are the temperatures and pressures required for fusion reactions to occur. It is also too hot for atoms to exist.
At room temperature on Earth, a hydrogen atom consists of a single proton surrounded by a single electron. If the volume of a hydrogen atom is represented by a large basketball stadium, the proton would be about the size of a bumble bee at the center, and the electron would be the size of a mote of dust floating around the edges. But within the Sun, the temperature is very high and particles are moving at very high speeds, smashing into each other with a great amount of kinetic energy. The electrons are quickly knocked free, destroying the atoms, and creating a “soup” of protons and electrons called a plasma.
In the plasma at the Sun’s core, colliding protons can fuse. Fusion is actually a multi-step process in which four protons become a helium nucleus that weighs a little less than the four protons. In the process, other particles, such as photons and neutrinos are released. A photon has no mass. It is a form of pure energy that travels through space. As we’ll see later in this chapter, light, x-rays, and radio waves are all composed of photons.
When a photon released by the fusion reaction strikes a particle, its energy is absorbed and almost immediately given off as another photon. That photon then hits another particle, and on and on. Each photon travels at the speed of light, but it only travels about a millimeter before it hits another particle. After each collision, the new photon that results may go in any direction—outwards towards the surface of the Sun, sideways, or even back into the center. A bit of energy will travel in this manner for an average of ten million years, before finally reaching the upper level of the Sun. The region above the core, where photons make their long wandering journeys, is called the radiation zone.
Eventually, the photons reach the upper 15% of the Sun, where the temperature and pressure have fallen enough for atoms to exist. Here, electrons can join with nuclei to produce atoms. Atoms absorb the energy of the photons and huge volumes of hydrogen gas just above the radiation zone heat up and start to float upwards, with cooler gas falling to take its place. We have reached the convection zone, where the Sun’s energy flows in huge convection currents.
The tops of the convective cells can be seen in telescope photographs of the Sun’s surface. Each one is about the size of Wyoming , and appears a little lighter than the region between the cells, where material has cooled and is beginning to sink. The overall effect is like a bowl of rice grains, and is called granulation. We see the hot centers of the grains because the atoms give off photons as they cool—photons of light, as well as ultraviolet and infrared photons—which bathe our planet in life-giving energy.
V. Space Weather and Space Storms
…a visit to the Space Science Lab at University of California Berkeley.
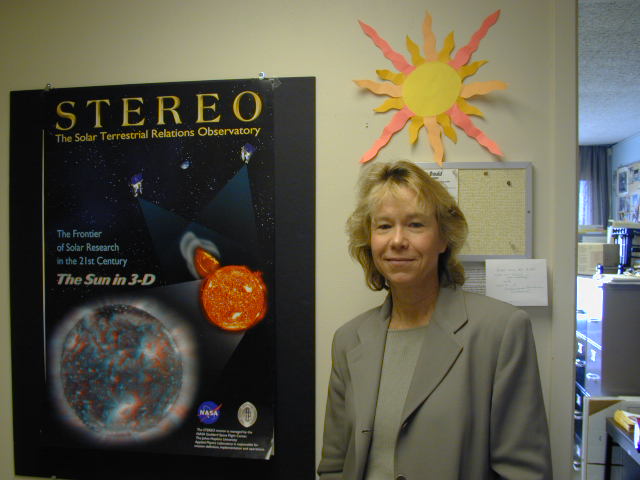
The Sun’s surface is a seething cauldron of gases and photons. Material spews off the Sun’s surface in copious quantities. The whole region of space around the Sun is affected, all the way to the Earth and even the outermost planets. NASA’s Office of Space Science (OSS) has a whole division called the “Sun-Earth Connection” that studies the Sun and “space weather.” The NASA TRACE spacecraft (Transition Region and Coronal Explorer) has captured images and movies of the Sun’s surface that show in exquisite detail the violence of activity in the Sun’s atmosphere and surface.
Working on NASA’s Sun-Earth Connection program, studying space weather, is Dr. Janet Luhmann, at University of California’s Space Science Laboratory in Berkeley. We visit her to find out what space weather means to us here on Earth.
Dr. Luhmann tells us, “Space weather is weather above the atmosphere of the Earth. We are familiar with dangerous aspects of weather in Earth’s atmosphere from high winds, thunderstorms, hailstorms, tornadoes, and hurricanes. The space above Earth’s atmosphere is not really a vacuum. It is filled with particles and magnetic fields coming from both the Earth itself and the Sun. When we are far enough from the Earth the Sun’s influence takes over, and we are in what is called the solar wind. The solar wind fills the solar system, so we are effectively immersed in the Sun’s outer atmosphere, as are Mercury, Venus, Mars, and all the planets. While the solar wind, which is made of ionized gas or plasma, is nowhere near as dense as wind in the Earth’s atmosphere, it still poses dangers to us.
“We often observe violent events on the Sun. For example flares are regions where sudden energetic eruptions happen. They last from minutes to hours and can give off huge amounts of particles and radiation including energetic particles, X-rays, radio waves, and ultraviolet light. But it’s not flares that cause most problems on Earth. Coronal mass ejections (CMEs) are gigantic blobs of the Sun’s upper atmosphere, or corona, that explode into space, sometimes in our direction. The size of CMEs is often a large fraction of the diameter of the Sun, but they grow still larger as they expand outward into the solar wind. We cannot see CMEs with our naked eyes unless something like a total solar eclipse, or a special device called a coronagraph, blocks the bright solar surface and lets us see the faint coronal light. The leading edges of fast-moving CMEs drive giant shock waves before them as they move through the solar wind at speeds up to 2000 km per second. In one CME, as much as 10 billion tons of the Sun’s atmosphere can be blown into interplanetary space.
“The solar wind is not uniform and constant-there are often ‘gusts’ and big changes in plasmas and magnetic fields. The CME makes an especially strong gust that acts like a snow plow in the solar wind. If the shock wave, the snow-plowed solar wind, and the coronal blob hit the Earth’s magnetic field, they distort it and disturb the space close to the Earth, including our own upper atmosphere. The CME-caused shock wave also adds a lot of energy to a few of the solar wind particles, as well as to some of the particles in the space around the Earth, increasing the radiation levels at high altitudes. The Sun has its own version of seasons- the frequency of sunspots, flares, and CMEs fluctuates on an 11-year cycle between times of maximum activity and minimum activity.
“CMEs can trigger severe magnetic storms near Earth and wreak havoc with spacecraft components, satellite communications systems, and multi-use space networks such as global positioning satellite (GPS) system. Onboard satellite computer chips can get zapped and then false commands occur due to data bits that have flipped their binary value. Satellite solar cells get damaged and their performance degraded from radiation. The disturbed ionosphere interferes with ground communications to satellites, and solar radio noise is received by antennas meant for our own radio communications. When a severe magnetic storm from a CME hits Earth, huge electric currents can be produced in our upper atmosphere causing it to heat up and expand. This in turn increases the atmospheric friction or drag on satellites in orbit and causes their orbits to decay (decrease in altitude) more rapidly than usual. On Earth’s surface, at high latitudes, there can even be electric currents set up in electric power transformers and oil pipelines, resulting in overload and failure of power grids or increased corrosion in the pipelines. Of course, there is also direct threat to the health of astronauts onboard the Space Shuttle and the International Space Station, due to the increased radiation near Earth around the times of magnetic storms. Energetic particles can also leak into Earth’s polar regions and expose people in high flying aircraft on polar routes to increased radiation-equivalent to a couple of chest X-rays.
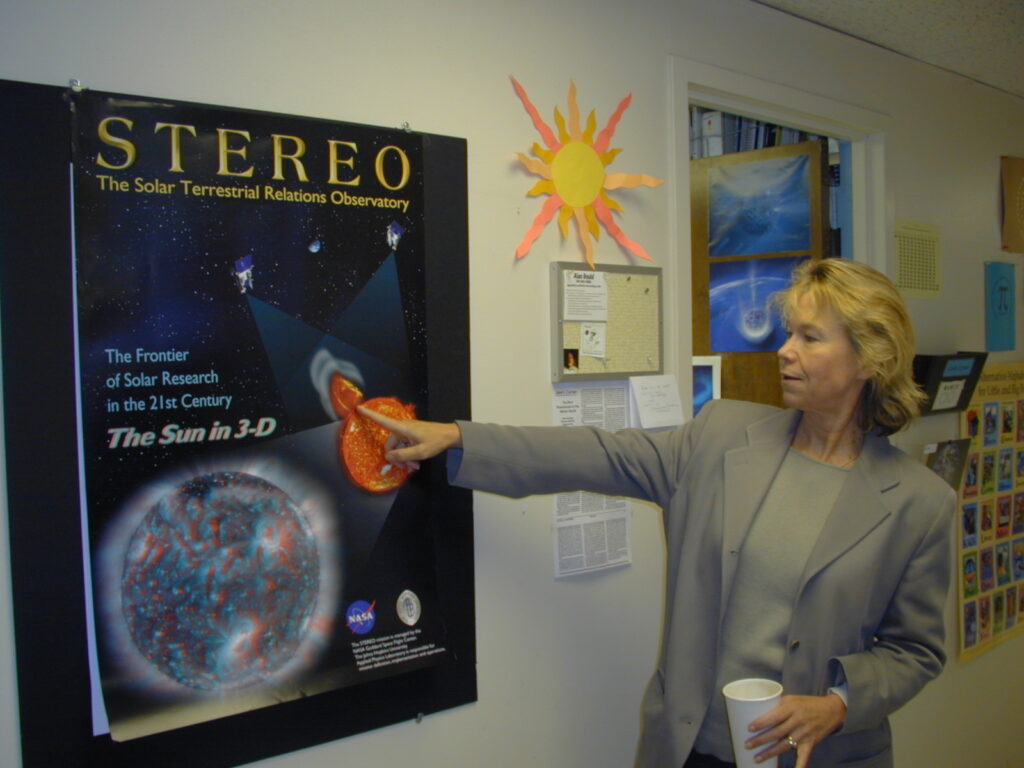
on an image of the Sun.
“Not all solar eruptions will hit Earth. A mass ejection may look very threatening in a coronagraph image but not produce anything at Earth if it is moving away from us or off to the side. Sometimes energetic particles produced by the CME-caused shock wave in the solar wind reach us days before the shock itself. These are sometimes a good indication that we will be hit, but the severity of the following geomagnetic storm is hard to tell from these particles alone.”
Dr. Luhmann explains to us about the latest Sun-Earth Connection mission that she is a Principal Investigator on:
“Despite the importance of CMEs, they are not well understood. While CMEs are now seen routinely with existing instruments, those erupting directly towards us are least likely to be detected. Until their 3-D structure is revealed, we cannot be certain of their nature, behavior, and origins. Our goal is to predict when there will be bad episodes of space weather-storms that can cause power blackouts and endanger airplane landings because of GPS navigation problems. There are only a few such bad episodes each solar cycle, and though there are more at the time of solar cycle activity maximum, bad storms can happen any time during the solar cycle. Predicting space weather is in its infancy and it’s very difficult. For example, a large CME was observed in the corona in June of 2000 and we expected a humongous magnetic storm, but it didn’t happen. Normally, for material from a solar eruption to reach Earth takes about four days. But a fast ejection can take as little as two days to reach Earth, and the first effects of an approaching shock can happen within an hour of the eruption from the Sun.
“To study solar eruptions in 3-D, we are preparing a new spacecraft mission called STEREO, for Solar Terrestrial Observatory. STEREO will include two spacecraft, both orbiting the Sun in the same orbit as Earth, but one leading ahead of Earth and one lagging behind Earth in its orbit. This will give us a stereoscopic 3-D view of the Sun, with ‘eyes’ separated by millions of miles. The craft will slowly drift apart through the two to five year mission which is set to launch in 2004. In addition to solar and coronal imagers, the STEREO spacecraft will carry magnetometers to detect the solar wind’s magnetic field as well as plasma sensors to detect the solar wind particles themselves. Here at the Space Sciences Lab in Berkeley, we are developing the sensors for those invisible parts: the solar wind plasma and magnetic fields. When STEREO observations are combined with data from instruments on Earth or in low Earth orbit, the buildup of magnetic energy, eruption, trajectory, and structure of Earthward-bound CMEs can be tracked in three dimensions, ‘from cradle to grave’.”
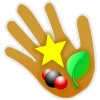
EF4.1. Investigation: Space Weather Now
Visit https://spaceweather.com/ to find out the latest “space weather forcasts” as well as news about space weather.
VI. Conclusion
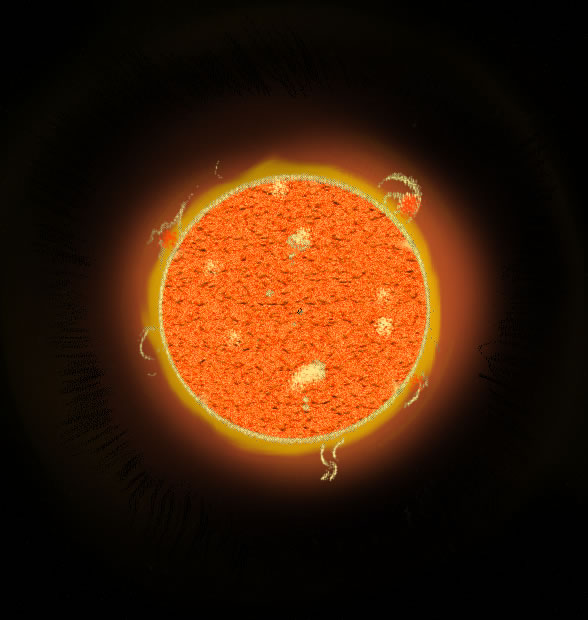
In this chapter we traced the energy that provides heat and light on Earth back to its origin in the center of the Sun. We also followed the flow of that energy from the center of the Sun to the surface where it emerges into space and saw what happens to that energy as it approaches the vicinity of Earth. In the next chapter, we take a closer look at the energy itself, and answer the question, “What is light?”